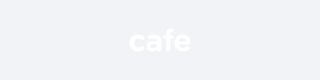
We studied the nervous system at a cellular level in chapter 12.
In these next two chapters, we move up the structural hierarchy to study the nervous system at the organ and system levels of organization. The spinal cord is an “information highway” between your brain and your trunk and limbs. It is about as thick as a finger, and extends through the vertebral canal as far as your first lumbar vertebra. At regular intervals, it gives off a pair of spinal nerves that receive sensory input from the skin, muscles, bones, joints, and viscera, and that issue motor commands back to muscle and gland cells.
The spinal cord is a component of the central nervous system and the spinal nerves a component of the peripheral nervous system, but these central and peripheral components are so closely linked structurally and functionally that it is appropriate that we consider them together in this chapter. The brain and cranial
nerves will be discussed in chapter 14.
The Spinal Cord
Objectives
When you have completed this section, you should be able to
• name the two types of tissue in the central nervous system and state their locations;
• describe the gross and microscopic anatomy of the spinal cord; and
• name the major conduction pathways of the spinal cord and state their functions.
Functions
The spinal cord serves three principal functions:
1. Conduction.
The spinal cord contains bundles of nerve fibers that conduct information up and down the cord, connecting different levels of the trunk with each other and with the brain. This enables sensory information to reach the brain, motor commands to reach the effectors, and input received at one level of the cord to affect output from another level.
2. Locomotion.
Walking involves repetitive, coordinated contractions of several muscle groups in the limbs. Motor neurons in the brain initiate walking and determine its speed, distance, and direction, but the simple repetitive muscle contractions that put one foot in front of another, over and over, are coordinated by groups of neurons called central pattern generators in the cord. These neuronal circuits produce the sequence of outputs to the extensor and flexor muscles that cause alternating movements of
the legs.
3. Reflexes.
Reflexes are involuntary stereotyped responses to stimuli. They involve the brain, spinal cord, and peripheral nerves.
Gross Anatomy
The spinal cord (fig. 13.1) is a cylinder of nervous tissue that begins at the foramen magnum and passes through the vertebral canal as far as the inferior margin of the first lumbar vertebra (L1). In adults, it averages about 1.8 cm thick and 45 cm long. Early in fetal development, the spinal cord extends for the full length of the vertebral column.
However, the vertebral column grows faster than the spinal cord, so the cord extends only to L3 by the time of birth and to L1 in an adult. Thus, it occupies only the upper two-thirds of the vertebral canal; the lower one-third is described shortly. The cord gives rise to 31 pairs of spinal nerves that pass through the intervertebral foramina. Although the spinal cord is not visibly segmented, the part supplied by each pair of spinal nerves is called a segment.
The cord exhibits longitudinal grooves on its ventral and dorsal sides—the ventral median fissure and dorsal median sulcus, respectively. The spinal cord is divided into cervical, thoracic, lumbar, and sacral regions. It may seem odd that it has a
sacral region when the cord itself ends well above the sacrum. These regions, however, are named for the level of the vertebral column from which the spinal nerves emerge, not for the vertebrae that contain the cord itself.
In the inferior cervical region, a cervical enlargement of the cord gives rise to nerves of the upper limbs. In the lumbosacral region, there is a similar lumbar enlargement where nerves to the pelvic region and lower limbs arise. Inferior to the lumbar enlargement, the cord tapers to a point called the medullary cone. The lumbar enlargement and medullary cone give rise to a bundle of nerve roots that occupy the canal of vertebrae L2 to S5. This bundle, named the cauda equina1 (CAW-duh ee-KWY-nah) for its resemblance to a horse’s tail, innervates the pelvic organs and lower limbs.
Meninges of the Spinal Cord
The spinal cord and brain are enclosed in three fibrous membranes called meninges (meh-NIN-jeez)—singular, meninx2 (MEN-inks). These membranes separate the soft tissue of the central nervous system from the bones of the vertebrae and skull. From superficial to deep, they are the dura mater, arachnoid mater, and pia mater. The dura mater3 (DOO-ruh MAH-tur) forms a loose fitting sleeve called the dural sheath around the spinal cord. It is a tough collagenous membrane with a thickness and texture similar to a rubber kitchen glove. The space between the sheath and vertebral bone, called the epidural
space, is occupied by blood vessels, adipose tissue, and loose connective tissue (fig. 13.2a ).
Anesthetics are sometimes introduced to this space to block pain signals during childbirth or surgery; this procedure is called epidural anesthesia. The arachnoid4 (ah-RACK-noyd) mater adheres to the dural sheath. It consists of a simple squamous epithelium, the arachnoid membrane, adhering to the inside of the dura, and a loose mesh of collagenous and elastic fibers spanning the gap between the arachnoid membrane and the pia mater.
This gap, called the subarachnoid space, is filled with cerebrospinal fluid (CSF), a clear liquid discussed in chapter 14.
The pia5 (PEE-uh) mater is a delicate, translucent membrane that closely follows the contours of the spinal cord. It continues beyond the medullary cone as a fibrous strand, the terminal filum, forming part of the coccygeal ligament that anchors the cord to vertebra L2. At regular intervals along the cord, extensions of the pia called denticulate ligaments extend through the arachnoid to the dura, anchoring the cord and preventing side-to-side movements.
Cross-Sectional Anatomy
Figure 13.2a shows the relationship of the spinal cord to a vertebra and spinal nerve, and figure 13.2b shows the cord
itself in more detail. The spinal cord, like the brain, consists of two kinds of nervous tissue called gray and white
matter. Gray matter has a relatively dull color because it
contains little myelin. It contains the somas, dendrites,
and proximal parts of the axons of neurons. It is the site of
synaptic contact between neurons, and therefore the site
of all synaptic integration (information processing) in the
central nervous system. White matter contains an abundance
of myelinated axons, which give it a bright, pearly
white appearance. It is composed of bundles of axons,
called tracts, that carry signals from one part of the CNS to
another. In fixed and silver-stained nervous tissue, gray
matter tends to have a darker brown or golden color and
white matter a lighter tan to yellow color.
Gray Matter
The spinal cord has a central core of gray matter that looks somewhat butterfly- or H-shaped in cross sections. The core consists mainly of two dorsal (posterior) horns, which extend toward the dorsolateral surfaces of the cord, and two thicker ventral (anterior) horns, which extend toward the ventrolateral surfaces. The right and left sides are connected by a gray commissure. In the middle of the commissure is the central canal, which is collapsed in most areas of the adult spinal cord, but in some places (and in young children) remains open, lined with ependymal cells, and filled with CSF.
As a spinal nerve approaches the cord, it branches
into a dorsal root and ventral root. The dorsal root carries
sensory nerve fibers, which enter the dorsal horn of the
cord and sometimes synapse with an interneuron there.
Such interneurons are especially numerous in the cervical
and lumbar enlargements and are quite evident in histological
sections at these levels. The ventral horns contain
the large somas of the somatic motor neurons. Axons from
these neurons exit by way of the ventral root of the spinal
nerve and lead to the skeletal muscles. The spinal nerve
roots are described more fully later in this chapter.
In the thoracic and lumbar regions, an additional lateral
horn is visible on each side of the gray matter. It contains
neurons of the sympathetic nervous system, which
send their axons out of the cord by way of the ventral root
along with the somatic efferent fibers.
White Matter
The white matter of the spinal cord surrounds the gray matter and consists of bundles of axons that course up and down the cord and provides avenues of communication between different levels of the CNS. These bundles are arranged in three pairs called columns or funiculi8 (few-NIC-you-lie)—a dorsal (posterior), lateral, and ventral (anterior) column on each side. Each column consists of subdivisions called tracts or fasciculi9 (fah-SIC-you-lye).
Spinal Tracts
Knowledge of the locations and functions of the spinaltracts is essential in diagnosing and managing spinal cord injuries. Ascending tracts carry sensory information up the cord and descending tracts conduct motor impulses down. All nerve fibers in a given tract have a similar origin, destination, and function.
Several of these tracts undergo decussation10 (DEEcuh-
SAY-shun) as they pass up or down the brainstem and
spinal cord—meaning that they cross over from the left
side of the body to the right, or vice versa. As a result, the
left side of the brain receives sensory information from the
right side of the body and sends its motor commands to
that side, while the right side of the brain senses and controls
the left side of the body. A stroke that damages motor
centers of the right side of the brain can thus cause paralysis
of the left limbs and vice versa. When the origin and
destination of a tract are on opposite sides of the body, we
say they are contralateral11 to each other. When a tract
does not decussate, so the origin and destination of its
fibers are on the same side of the body, we say they are
ipsilateral.12
The major spinal cord tracts are summarized in table 13.1 and figure 13.4. Bear in mind that each tract is repeated on the right and left sides of the spinal cord.
Ascending Tracts
Ascending tracts carry sensory signals up the spinal cord. Sensory signals typically travel across three neurons from their origin in the receptors to their destination in the sensory areas of the brain: a first-order neuron that detects a stimulus and transmits a signal to the spinal cord or brainstem; a second-order neuron that continues as far as a “gateway” called the thalamus at the upper end of the brainstem; and a third-order neuron that carries the signal the rest of the way to the sensory region of the cerebral cortex.
The axons of these neurons are called the firstthrough
third-order nerve fibers. Deviations from the pathway
described here will be noted for some of the sensory
systems to follow.
The major ascending tracts are as follows. The names
of most ascending tracts consist of the prefix spino- followed
by a root denoting the destination of its fibers in the brain.
• The gracile13 fasciculus (GRAS-el fah-SIC-you-lus) carries signals from the midthoracic and lower parts of the body. Below vertebra T6, it composes the entire dorsal column. At T6, it is joined by the cuneate fasciculus, discussed next. It consists of first-order nerve fibers that travel up the ipsilateral side of the spinal cord and terminate at the gracile nucleus in the
medulla oblongata of the brainstem. These fibers carry signals for vibration, visceral pain, deep and discriminative touch (touch whose location one can
precisely identify), and especially proprioception14
from the lower limbs and lower trunk. (Proprioception
is a nonvisual sense of the position and movements of
the body.)
• The cuneate15 (CUE-nee-ate) fasciculus (fig. 13.5a ) joins the gracile fasciculus at the T6 level. It occupies the lateral portion of the dorsal column and forces the gracile fasciculus medially. It carries the same type of sensory signals, originating from level T6 and up (from the upper limb and chest). Its fibers end in the cuneate nucleus on the ipsilateral side of the medulla oblongata. In the medulla, second-order fibers of the
gracile and cuneate systems decussate and form the
medial lemniscus16 (lem-NIS-cus), a tract of nerve
fibers that leads the rest of the way up the brainstem
to the thalamus. Third-order fibers go from the
thalamus to the cerebral cortex. Because of
decussation, the signals carried by the gracile and
cuneate fasciculi ultimately go to the contralateral
cerebral hemisphere.
• The spinothalamic (SPY-no-tha-LAM-ic) tract
(fig. 13.5b ) and some smaller tracts form the
anterolateral system, which passes up the anterior
and lateral columns of the spinal cord. The
spinothalamic tract carries signals for pain,
temperature, pressure, tickle, itch, and light or crude
touch. Light touch is the sensation produced by
stroking hairless skin with a feather or cotton wisp,
without indenting the skin; crude touch is touch
whose location one can only vaguely identify. In this
pathway, first-order neurons end in the dorsal horn of
the spinal cord near the point of entry. Second-order
neurons decussate to the opposite side of the spinal
cord and there form the ascending spinothalamic
tract. These fibers lead all the way to the thalamus.
Third-order neurons continue from there to the
cerebral cortex.
• The dorsal and ventral spinocerebellar (SPY-no- SERR-eh-BEL-ur) tracts travel through the lateral column and carry proprioceptive signals from the limbs and trunk to the cerebellum, a large motor control area at the rear of the brain. The first-order neurons of this system originate in the muscles and tendons and end in the dorsal horn of the spinal cord. Second-order neurons send their fibers up the spinocerebellar tracts and end in the cerebellum.
Fibers of the dorsal tract travel up the ipsilateral side of the spinal cord. Those of the ventral tract cross over and travel up the contralateral side but then cross back in the brainstem to enter the ipsilateral cerebellum. Both tracts provide the cerebellum with feedback needed to coordinate muscle action, as discussed in chapter 14.
Tracts of the Spinal Cord
Ascending Pathways of the CNS.
Descending Tracts
Descending tracts carry motor signals down the brainstem and spinal cord. A descending motor pathway typically involves two neurons called the upper and lower motor neuron. The upper motor neuron begins with a soma in the cerebral cortex or brainstem and has an axon that terminates on a lower motor neuron in the brainstem or spinal cord. The axon of the lower motor neuron then leads the rest of the way to the muscle or other target organ. The names of most descending tracts consist of a word root denoting the point of origin in the brain, followed by the suffix -spinal. The major descending tracts are described here.
• The corticospinal (COR-tih-co-SPY-nul) tracts carry motor signals from the cerebral cortex for precise, finely coordinated limb movements. The fibers of this system form ridges called pyramids on the ventral surface of the medulla oblongata, so these tracts were once called pyramidal tracts. Most corticospinal fibers decussate in the lower medulla and form the lateral
corticospinal tract on the contralateral side of the spinal cord. A few fibers remain uncrossed and form the ventral corticospinal tract on the ipsilateral side (fig. 13.6). Fibers of the ventral tract decussate lowerin the spinal cord, however, so even they control contralateral muscles.
• The tectospinal (TEC-toe-SPY-nul) tract begins in a midbrain region called the tectum and crosses to the contralateral side of the brainstem. In the lower medulla, it branches into lateral and medial tectospinal tracts of the upper spinal cord. These are involved in reflex movements of the head, especially in response to visual and auditory stimuli.
• The lateral and medial reticulospinal (reh-TIC-you-lo- SPY-nul) tracts originate in the reticular formation of the brainstem. They control muscles of the upper and lower limbs, especially to maintain posture and balance. They also contain descending analgesic pathways that reduce the transmission of pain signals to the brain (see chapter 16).
• The vestibulospinal (vess-TIB-you-lo-SPY-nul) tract begins in a brainstem vestibular nucleus that receives impulses for balance from the inner ear. The tract passes down the ventral column of the spinal cord and controls limb muscles that maintain balance and posture.
Rubrospinal tracts are prominent in other mammals, where they aid in muscle coordination. Although often pictured in illustrations of human anatomy, they are almost nonexistent in humans and have little functional importance.
Two Descending Pathways of the CNS.
Insight 13.2 Clinical Application
Poliomyelitis and Amyotrophic Lateral Sclerosis
Poliomyelitis17 and amyotrophic lateral sclerosis18 (ALS) are two diseases that involve destruction of motor neurons. In both diseases, the skeletal muscles atrophy from lack of innervation. Poliomyelitis is caused by the poliovirus, which destroys motor neurons in the brainstem and ventral horn of the spinal cord. Signs of polio include muscle pain, weakness, and loss of some reflexes, followed by paralysis, muscular atrophy, and sometimes respiratory arrest. The virus spreads by fecal contamination of water. Historically, polio afflicted mainly children, who sometimes contracted the virus in the summer by swimming in contaminated pools. The polio vaccine has nearly eliminated new cases.
ALS is also known as Lou Gehrig disease after the baseball player who contracted it. It is marked not only by the degeneration of motor neurons and atrophy of the muscles, but also sclerosis of the lateral regions of the spinal cord—hence its name. In most cases of ALS, neurons are destroyed by an inability of astrocytes to reabsorb glutamate from the tissue fluid, allowing this neurotransmitter to accumulate to a toxic level. The early signs of ALS include muscular weakness and difficulty in
speaking, swallowing, and using the hands. Sensory and intellectual functions remain unaffected, as evidenced by the accomplishments of astrophysicist and best-selling author Stephen Hawking, who was stricken with ALS while he was in college. Despite near-total paralysis, he remains highly productive and communicates with the aid of a speech synthesizer and computer. Tragically, many people are quick to assume that those who have lost most of their ability to communicate their ideas
and feelings have no ideas and feelings to communicate. To a victim, this may be more unbearable than the loss of motor function itself.
The Spinal Nerves
Objectives
When you have completed this section, you should be able to
• describe the attachment of a spinal nerve to the spinal cord;
• trace the branches of a spinal nerve distal to its attachment;
• name the five plexuses of spinal nerves and describe their general anatomy;
• name some major nerves that arise from each plexus; and
• explain the relationship of dermatomes to the spinal nerves.
General Anatomy of Nerves and Ganglia
The spinal cord communicates with the rest of the body by way of the spinal nerves. Before we discuss those specific nerves, however, it is necessary to be familiar with the structure of nerves and ganglia in general. A nerve is a cordlike organ composed of numerous nerve fibers (axons) bound together by connective tissue (fig. 13.8). If we compare a nerve fiber to a wire carrying an electrical current in one direction, a nerve would be comparable to an electrical cable composed of thousands of wires carrying currents in opposite directions. A nerve contains anywhere from a few nerve fibers to more than a million. Nerves usually have a pearly white color and resemble frayed string as they divide into smaller and smaller branches.
Anatomy of a Nerve
Nerve fibers of the peripheral nervous system are ensheathed in Schwann cells, which form a neurilemma and often a myelin sheath around the axon (see chapter 12). External to the neurilemma, each fiber is surrounded by a basal lamina and then a thin sleeve of loose connective tissue called the endoneurium. In most nerves, the nerve fibers are gathered in bundles called fascicles, each wrapped in a sheath called the perineurium. The perineurium is composed of one to six layers of overlapping, squamous, epithelium-like cells. Several fascicles are then bundled together and wrapped in an outer epineurium to compose the nerve as a whole. The epineurium is composed of dense irregular fibrous connective tissue and protects
the nerve from stretching and injury. Nerves have a high metabolic rate and need a plentiful blood supply. Blood vessels penetrate as far as the perineurium, and oxygen and nutrients diffuse through the extracellular fluid from there to the nerve fibers.
Think About It
How does the structure of a nerve compare to that of a skeletal muscle? Which of the descriptive terms for nerves have similar counterparts in muscle histology?
Peripheral nerve fibers are of two kinds: sensory (afferent) fibers carry signals from sensory receptors to the CNS, and motor (efferent) fibers carry signals from the CNS
to muscles and glands. Both sensory and motor fibers can
also be described as somatic or visceral and as general or
special depending on the organs they innervate (table 13.2).
A mixed nerve consists of both sensory and motor
fibers and thus transmits signals in two directions,
although any one nerve fiber within the nerve transmits
signals one way only. Most nerves are mixed. Purely
sensory nerves, composed entirely of sensory axons, are
less common; they include the olfactory and optic
nerves discussed in chapter 14. Nerves that carry only
motor fibers are called motor nerves. Many nerves often
described as motor are actually mixed because they
carry sensory signals of proprioception from the muscle
back to the CNS.
If a nerve resembles a thread, a ganglion19 resembles
a knot in the thread. A ganglion is a cluster of cell
bodies (somas) outside the CNS. It is enveloped in an
epineurium continuous with that of the nerve. Among
the somas are bundles of nerve fibers leading into and out
of the ganglion. Figure 13.9 shows a type of ganglion
called the dorsal root ganglion associated with the spinal
nerves.
Spinal Nerves
There are 31 pairs of spinal nerves: 8 cervical (C1–C8), 12 thoracic (T1–T12), 5 lumbar (L1–L5), 5 sacral (S1–S5), and
1 coccygeal (Co) (fig. 13.10). The first cervical nerve emerges between the skull and atlas, and the others emerge through intervertebral foramina, including the anterior and posterior foramina of the sacrum.
Proximal Branches
Each spinal nerve has two points of attachment to the spinal cord (fig. 13.11). Dorsally, a branch of the spinal nerve called the dorsal root divides into six to eight nerve rootlets that enter the spinal cord (fig. 13.12). A little distal to the rootlets is a swelling called the dorsal root ganglion, which contains the somas of afferent neurons. Ventrally, another row of six to eight rootlets leave the spinal cord and converge to form the ventral root.
The dorsal and ventral roots merge, penetrate the dural sac, enter the intervertebral foramen, and there form the spinal nerve proper. Spinal nerves are mixed nerves, with a two-way traffic of afferent (sensory) and efferent (motor) signals. Afferent
signals approach the cord by way of the dorsal root and enter the dorsal horn of the gray matter. Efferent signals begin at the somas of motor neurons in the ventral horn and leave the spinal cord via the ventral root. Some viruses invade the central nervous system by way of these roots (see insight 13.3).
The dorsal and ventral roots are shortest in the cervical region and become longer inferiorly. The roots that arise from segments L2 to Co of the cord form the cauda equina.
Distal Branches
Distal to the vertebrae, the branches of a spinal nerve are more complex (fig. 13.13). Immediately after emerging from the intervertebral foramen, the nerve divides into a dorsal ramus,20 a ventral ramus, and a small meningeal branch. The meningeal branch (see fig. 13.11) reenters the vertebral canal and innervates the meninges, vertebrae, and spinal ligaments. The dorsal ramus innervates the muscles and joints in that region of the spine and the skin of the back. The ventral ramus innervates the ventral and lateral skin and muscles of the trunk and gives rise to nerves of the limbs.
Anatomy of a Ganglion. The dorsal root ganglion contains the somas of unipolar sensory neurons conducting signals to the spinal cord. To the left of it is the ventral root of the spinal nerve, which conducts motor signals away from the spinal cord. (The ventral root is not part of the v ganglion.)
The Spinal Nerve Roots and Plexuses
Branches of a Spinal Nerve in Relation to the Spinal Cord and Vertebra
Figure 13.11 Branches of a Spinal Nerve in Relation to the Spinal Cord and Vertebra (cross section).
The Point of Entry of Two Spinal Nerves into the Spinal Cord
Figure 13.12 The Point of Entry of Two Spinal Nerves into the Spinal Cord. Dorsal view with vertebrae cut away. Note that each dorsal root divides into several rootlets that enter the spinal cord. A segment of the spinal cord is the portion receiving all the rootlets of one spinal nerve.
Rami of the Spinal Nerves.
Figure 13.13 Rami of the Spinal Nerves. (a) Anterolateral view of the spinal nerves and their subdivisions in relation to the spinal cord and vertebrae. (b) Cross section of the thorax showing innervation of muscles of the chest and back.
cervical plexus
Nerve Plexuses
Except in the thoracic region, the ventral rami branch and anastomose (merge) repeatedly to form five weblike nerve
plexuses: the small cervical plexus deep in the neck, the brachial plexus near the shoulder, the lumbar plexus of the lower back, the sacral plexus immediately inferior to this, and finally the tiny coccygeal plexus adjacent to the lower sacrum and coccyx. A general view of these plexuses is shown in figure 13.10; they are illustrated and described in tables 13.3 through 13.6. The muscle actions controlled by these nerves are described in the muscle tables in chapter 10.
Insight 13.4 Clinical Application
Spinal Nerve Injuries
The radial and sciatic nerves are especially vulnerable to injury. The radial nerve, which passes through the axilla, may be compressed against the humerus by improperly adjusted crutches, causing crutch paralysis. A similar injury often resulted from the now-discredited practice of trying to correct a dislocated shoulder by putting a foot in a person’s armpit and pulling on the arm. One consequence of radial nerve injury is wrist drop—the fingers, hand, and wrist are chronically flexed because the extensor muscles supplied by the radial nerve are paralyzed.
Because of its position and length, the sciatic nerve of the hip and thigh is the most vulnerable nerve in the body. Trauma to this nerve produces sciatica, a sharp pain that travels from the gluteal region along the posterior side of the thigh and leg as far as the ankle. Ninety percent of cases result from a herniated intervertebral disc or osteoarthritis of the lower spine, but sciatica can also be caused by pressure from a pregnant uterus, dislocation of the hip, injections in the wrong area of the buttock, or sitting for a long time on the edge of a hard chair. Men sometimes suffer sciatica from the habit of sitting on a wallet carried in the hip pocket.
brachial plexus
lumbar plexus
sacral and coccygeal plexus
Cutaneous Innervation and Dermatomes
Each spinal nerve except C1 receives sensory input from a specific area of skin called a dermatome.22 A dermatome
map (fig. 13.19) is a diagram of the cutaneous regions innervated by each spinal nerve. Such a map is oversimplified,
however, because the dermatomes overlap at their edges by as much as 50%. Therefore, severance of one sensory
nerve root does not entirely deaden sensation from a dermatome. It is necessary to sever or anesthetize three successive spinal nerves to produce a total loss of sensation from one dermatome. Spinal nerve damage is assessed by testing the dermatomes with pinpricks and noting areas in which the patient has no sensation.
dermatome
Before You Go On
Answer the following questions to test your understanding of the preceding section:
5. What is meant by the dorsal and ventral roots of a spinal nerve? Which of these is sensory and which is motor?
6. Where are the somas of the dorsal root located? Where are the somas of the ventral root?
7. List the five plexuses of spinal nerves and state where each one is located.
8. State which plexus gives rise to each of the following nerves: axillary, ilioinguinal, obturator, phrenic, pudendal, radial, and sciatic.
Somatic Reflexes
Objectives
When you have completed this section, you should be able to
• define reflex and explain how reflexes differ from othermotor actions;
• describe the general components of a typical reflex arc; and
• explain how the basic types of somatic reflexes function.
Most of us have had our reflexes tested with a little rubber hammer; a tap near the knee produces an uncontrollable jerk of the leg, for example. In this section, we discuss what reflexes are and how they are produced by an assembly of receptors, neurons, and effectors. We also survey the different types of neuromuscular reflexes and how they are important to motor coordination.
The Nature of Reflexes
Reflexes are quick, involuntary, stereotyped reactions of glands or muscles to stimulation. This definition sums up four important properties of a reflex:
1. Reflexes require stimulation—they are not spontaneous actions but responses to sensory input.
2. Reflexes are quick—they generally involve few if any interneurons and minimum synaptic delay.
3. Reflexes are involuntary—they occur without intent, often without our awareness, and they are difficult to suppress. Given an adequate stimulus, the response is essentially automatic. You may become conscious of the stimulus that evoked a reflex, and this awareness may enable you to correct or avoid a potentially dangerous situation, but awareness is not a part of the reflex itself. It may come after the reflex action has been completed, and somatic reflexes can occur even if the spinal cord has been severed so that no stimuli reach the brain.
4. Reflexes are stereotyped—they occur in essentially the same way every time; the response is very predictable.
Reflexes include glandular secretion and contractions of all three types of muscle. They also include some learned responses, such as the salivation of dogs in response to a sound they have come to associate with feeding time, first studied by Ivan Pavlov and named conditioned reflexes. In this section, however, we are concerned with unlearned skeletal muscle reflexes that are mediated by the brainstem and spinal cord. They result in the involuntary contraction of a muscle—for example, the quick withdrawal of your hand from a hot stove or the lifting of your foot when you step on something sharp. These are somatic reflexes, since they involve the somatic nervous system.
Chapter 15 concerns visceral reflexes. The somatic reflexes have traditionally been called spinal reflexes, although some visceral reflexes also involve the spinal cord, and some somatic reflexes are mediated more by the brain than by the spinal cord.
A somatic reflex employs a reflex arc, in which signals travel along the following pathway:
1. somatic receptors in the skin, a muscle, or a tendon;
2. afferent nerve fibers, which carry information from these receptors into the dorsal horn of the spinal cord;
3. interneurons, which integrate information; these are lacking from some reflex arcs;
4. efferent nerve fibers, which carry motor impulses to the skeletal muscles; and
5. skeletal muscles, the somatic effectors that carry out the response.
The Muscle Spindle
Many somatic reflexes involve stretch receptors in the muscles called muscle spindles. These are among the body’s proprioceptors —sense organs that monitor the position and movements of body parts. Muscle spindles are especially abundant in muscles that require fine control.
The hand and foot have 100 or more spindles per gram of muscle, whereas there are relatively few in large muscles with coarse movements and none at all in the middle-ear muscles. Muscle spindles provide the cerebellum with the feedback it needs to regulate the tension in the skeletal muscles.
Muscle spindles are about 4 to 10 mm long, tapered at the ends, and scattered throughout the fleshy part of a muscle (fig. 13.20). A spindle contains 3 to 12 modified muscle fibers and a few nerve fibers, all wrapped in a fibrous capsule. The muscle fibers within a spindle are called intrafusal23 fibers, while those of the rest of the muscle are called extrafusal fibers. Only the two ends of an intrafusal fiber have sarcomeres and are able to contract. The middle portion acts as the stretch receptor. There are two classes of intrafusal fibers: nuclear chain fibers, which have a single file of nuclei in the noncontractile region, and nuclear bag fibers, which are about twice as long and have nuclei clustered in a thick midregion.
Muscle spindles have three types of nerve fibers:
1. Primary afferent fibers, which end in annulospiral endings that coil around the middle of nuclear chain and nuclear bag fibers. These respond mainly to the onset of muscle stretch.
2. Secondary afferent fibers, which have flower-spray endings, somewhat resembling the dried head of a wildflower, wrapped primarily around the ends of the nuclear chain fibers. These respond mainly to prolonged stretch.
3. Gamma () motor neurons, which originate in the ventral horn of the spinal cord and lead to the contractile ends of the intrafusal fibers. The name distinguishes them from the alpha () motor neurons, which innervate the extrafusal fibers.
Gamma motor neurons adjust the tension in a muscle spindle to variations in the length of the muscle. When a muscle shortens, the motor neurons stimulate the ends of the intrafusal fibers to contract slightly. This keeps the intrafusal fibers taut and responsive at all times. Without this feedback, the spindles would become flabby when a skeletal muscle shortened. This feedback is clearly very important, because motor neurons constitute about one-third of all the motor fibers in a spinal nerve.
The Stretch Reflex
When a muscle is stretched, it “fights back”—it contracts, maintains increased tonus, and feels stiffer than an unstretched muscle. This response, called the stretch (myotatic24) reflex, helps to maintain equilibrium and posture. For example, if your head starts to tip forward, it stretches muscles such as the semispinalis and splenius capitis of the nuchal region (back of your neck). This stimulates their muscle spindles, which send afferent signals to the cerebellum by way of the brainstem. The cerebellum integrates this information and relays it to the cerebral cortex, and the cortex sends signals back to the nuchal muscles.
The muscles contract and raise your head. Stretch reflexes often feed back not to a single muscle but to a set of synergists and antagonists. Since the contraction of a muscle on one side of a joint stretches the antagonistic muscle on the other side, the flexion of a joint triggers a stretch reflex in the extensors, and extension stimulates a stretch reflex in the flexors. Consequently, stretch reflexes are valuable in stabilizing joints by balancing the tension of the extensors and flexors. They also
dampen (smooth) muscle action. Without stretch reflexes, a person’s movements tend to be jerky. Stretch reflexes are especially important in coordinating vigorous and precise movements such as dance.
A stretch reflex is mediated primarily by the brain and is not, therefore, strictly a spinal reflex, but a weak component of it is spinal and occurs even if the spinal cord is severed from the brain. The spinal component can be more pronounced if a muscle is stretched very suddenly. This occurs in a tendon reflex —the reflexive contraction of a muscle when its tendon is tapped, as in the familiar knee-jerk (patellar) reflex. Tapping the patellar ligament with a reflex hammer suddenly stretches the quadriceps femoris muscle of the thigh (fig. 13.21). This stimulates numerous muscle spindles in the quadriceps and sends an intense volley of signals to the spinal cord, mainly by way of primary afferent fibers.
In the spinal cord, the primary afferent fibers synapse directly with the motor neurons that return to the muscle, thus forming monosynaptic reflex arcs. That is, there is only one synapse between the afferent and efferent neuron, therefore little synaptic delay and a very prompt response. The motor neurons excite the quadriceps muscle, making it contract and creating the knee jerk.
There are many other tendon reflexes. A tap on the calcaneal tendon causes plantar flexion of the foot, a tap on the triceps brachii tendon causes extension of the elbow, and a tap on the cheek causes clenching of the jaw. Testing somatic reflexes is valuable in diagnosing many diseases that cause exaggeration, inhibition, or absence of reflexes, such as neurosyphilis, diabetes mellitus, multiple sclerosis, alcoholism, electrolyte imbalances, and lesions of the nervous system.
Stretch reflexes and other muscle contractions often
depend on reciprocal inhibition, a reflex phenomenon
that prevents muscles from working against each other by
inhibiting antagonists. In the knee jerk, for example, the
quadriceps femoris would not produce much joint movement
if its antagonists, the hamstring muscles, contracted
at the same time. But reciprocal inhibition prevents that
from happening. Some branches of the sensory fibers from
the muscle spindles in the quadriceps stimulate spinal
cord interneurons which, in turn, inhibit the motor neurons
of the hamstring muscles (fig. 13.21). The hamstring
muscles therefore remain relaxed and allow the quadriceps
to extend the knee.
The Flexor (Withdrawal) Reflex
A flexor reflex is the quick contraction of flexor muscles resulting in the withdrawal of a limb from an injurious stimulus. For example, suppose you are wading in a lake and step on a broken bottle with your right foot (fig. 13.22). Even before you are consciously aware of the pain, you quickly pull your foot away before the glass penetrates any deeper. This action involves contraction of the flexors and relaxation of the extensors in that limb; the latter is another case of reciprocal inhibition.
The protective function of this reflex requires more than a quick jerk like a tendon reflex, so it involves more complex neural pathways. Sustained contraction of the flexors is produced by a parallel after-discharge circuit in the spinal cord (see fig. 12.27, p. 473). This circuit is
part of a polysynaptic reflex arc—a pathway in which
signals travel over many synapses on their way back to
the muscle. Some signals follow routes with only a few
synapses and return to the flexor muscles quickly. Others
follow routes with more synapses, and therefore
more delay, so they reach the flexor muscles a little later.
Consequently, the flexor muscles receive prolonged output
from the spinal cord and not just one sudden stimu lus as in a stretch reflex. By the time these efferent signals
begin to die out, you will probably be consciously
aware of the pain and begin taking voluntary action to
prevent further harm.
The Crossed Extensor Reflex
In the preceding situation, if all you did was to quickly lift the injured leg from the lake bottom, you would fall over. To prevent this and maintain your balance, other reflexes shift your center of gravity over the leg that is still on the ground. The crossed extensor reflex is the contraction of extensor muscles in the limb opposite from the one that is withdrawn (fig. 13.22). It extends that limb and enables
you to keep your balance. To produce this reflex, branches
of the afferent nerve fibers cross from the stimulated side
of the body to the contralateral side of the spinal cord.
There, they synapse with interneurons, which, in turn,
excite or inhibit motor neurons to the muscles of the
contralateral limb.
In the ipsilateral leg (the side that was hurt), you
would contract your flexors and relax your extensors to lift
the leg from the ground. On the contralateral side, you
would relax your flexors and contract the extensors to
stiffen that leg, since it must suddenly support your entire
body. At the same time, signals travel up the spinal cord
and cause contraction of contralateral muscles of the hip
and abdomen to shift your center of gravity over the extended leg. To a large extent, the coordination of all
these muscles and maintenance of equilibrium is mediated
by the cerebellum and cerebral cortex.
The flexor reflex employs an ipsilateral reflex arc —
one in which the sensory input and motor output are on
the same sides of the spinal cord. The crossed extensor
reflex employs a contralateral reflex arc, in which the
input and output are on opposite sides. An intersegmental
reflex arc is one in which the input and output occur
at different levels (segments) of the spinal cord—for example,
when pain to the foot causes contractions of abdominal
and hip muscles higher up the body. Note that all of
these reflex arcs can function simultaneously to produce a
coordinated protective response to pain.
The Golgi Tendon Reflex
Golgi tendon organs are proprioceptors located in a tendon near its junction with a muscle (fig. 13.23). A tendon organ is about 1 mm long and consists of an encapsulated tangle of knobby nerve endings entwined in the collagen fibers of the tendon. As long as the tendon is slack, its collagen fibers are slightly spread and they put little pressure on the nerve endings woven among them. When muscle contraction pulls on the tendon, the collagen fibers come together like the two sides of a stretched rubber band and squeeze the nerve endings between them.
The nerve fiber sends signals to the spinal cord that provide
the CNS with feedback on the degree of muscle tension
at the joint.
The Golgi tendon reflex is a response to excessive
tension on the tendon. It inhibits motor neurons to the
muscle so the muscle does not contract as strongly. This
serves to moderate muscle contraction before it tears a tendon
or pulls it loose from the muscle or bone. Nevertheless,
strong muscles and quick movements sometimes
damage a tendon before the reflex can occur, causing such
athletic injuries as a ruptured calcaneal tendon.
The Golgi tendon reflex also functions when some
parts of a muscle contract more than others. It inhibits the
fibers connected with overstimulated tendon organs so
that their contraction is more comparable to the contraction
of the rest of the muscle. This reflex spreads the workload
more evenly over the entire muscle, which is beneficial
in such actions as maintaining a steady grip on a tool.
Table 13.7 and insight 13.5 describe some injuries
and other disorders of the spinal cord and spinal nerves.
Insight 13.5 Clinical Application
Spinal Cord Trauma
Each year in the United States, 10,000 to 12,000 people become paralyzed
by spinal cord trauma, usually as a result of vertebral fractures.
The group at greatest risk is males from 16 to 30 years old, because of
their high-risk behaviors. Fifty-five percent of their injuries are from
automobile and motorcycle accidents, 18% from sports, and 15% from
gunshot and stab wounds. Elderly people are also at above-average risk
because of falls, and in times of war, battlefield injuries account for
many cases.
Effects of Injury
Complete transection (severance) of the spinal cord causes immediate loss of motor control at and below the level of the injury. Transection superior to segment C4 presents a threat of respiratory failure. Victims also lose all sensation from the level of injury and below, although some patients temporarily feel burning pain within one or two dermatomes of the level of the lesion.
In the early stage, victims exhibit a syndrome (a suite of signs and
symptoms) called spinal shock. The muscles below the level of injury
exhibit flaccid paralysis and an absence of reflexes because of the lack
of stimulation from higher levels of the CNS. For 8 days to 8 weeks
after the accident, the patient typically lacks bladder and bowel
reflexes and thus retains urine and feces. Lacking sympathetic stimulation
to the blood vessels, a patient may exhibit neurogenic shock in
which the vessels dilate and blood pressure drops dangerously low.
Fever may occur because the hypothalamus cannot induce sweating to cool the body. Spinal shock can last from a few days to 3 months, but
typically lasts 7 to 20 days.
As spinal shock subsides, somatic reflexes begin to reappear, at
first in the toes and progressing to the feet and legs. Autonomic
reflexes also reappear. Contrary to the earlier urinary and fecal retention,
a patient now has the opposite problem, incontinence, as the
rectum and bladder empty reflexively in response to stretch. Both the
somatic and autonomic nervous systems typically exhibit exaggerated
reflexes, a state called hyperreflexia or the mass reflex reaction.
Stimuli such as a full bladder or cutaneous touch can trigger an
extreme cardiovascular reaction. The systolic blood pressure, normally
about 120 mmHg, jumps to as high as 300 mmHg. This causes
intense headaches and sometimes a stroke. Pressure receptors in the
major arteries sense this rise in blood pressure and activate a reflex
that slows the heart, sometimes to a rate as low as 30 or 40
beats/minute (bradycardia), compared to a normal rate of 70 to 80.
The patient may also experience profuse sweating and blurred vision.
Men at first lose the capacity for erection and ejaculation. They may
recover these functions later and become capable of climaxing and
fathering children, but without sexual sensation. In females, menstruation
may become irregular or cease.
The most serious permanent effect of spinal cord trauma is paralysis.
The flaccid paralysis of spinal shock later changes to spastic
paralysis as spinal reflexes are regained, but lack inhibitory control
from the brain. Spastic paralysis typically starts with chronic flexion
of the hips and knees (flexor spasms) and progresses to a state in
which the limbs become straight and rigid (extensor spasms). Three
forms of muscle paralysis are paraplegia, a paralysis of both lower
limbs resulting from spinal cord lesions at levels T1 to L1; quadriplegia,
the paralysis of all four limbs resulting from lesions above level
C5; and hemiplegia, paralysis of one side of the body, resulting not
from spinal cord injuries but usually from a stroke or other brain
lesion. Spinal cord lesions from C5 to C7 can produce a state of partial
quadriplegia—total paralysis of the lower limbs and partial paralysis
(paresis, or weakness) of the upper limbs.
Pathogenesis
Spinal cord trauma produces two stages of tissue destruction. The first is instantaneous—the destruction of cells by the traumatic event itself. The second wave of destruction, involving tissue death by necrosis and apoptosis, begins in minutes and lasts for days. It is far more destructive than the initial injury, typically converting a lesion in one spinal cord segment to a lesion that spans four or five segments, two above and two below the original site.
Microscopic hemorrhages appear in the gray matter and pia mater
within minutes and grow larger over the next 2 hours. The white matter
becomes edematous (swollen). This hemorrhaging and edema
spread to adjacent segments of the cord, and can fatally affect respiration
or brainstem function when it occurs in the cervical region.
Ischemia (iss-KEE-me-uh), the lack of blood, quickly leads to tissue
necrosis. The white matter regains circulation in about 24 hours, but
the gray matter remains ischemic. Inflammatory cells (leukocytes and
macrophages) infiltrate the lesion as the circulation recovers, and while
they clean up necrotic tissue, they also contribute to the damage by
releasing destructive free radicals and other toxic chemicals. The
necrosis worsens, and is accompanied by another form of cell death,
apoptosis (see chapter 5). Apoptosis of the spinal oligodendrocytes, the
myelinating glial cells of the CNS, results in demyelination of spinal
nerve fibers, followed by death of the neurons.
In as little as 4 hours, this second wave of destruction, called posttraumatic
infarction, consumes about 40% of the cross-sectional area
of the spinal cord; within 24 hours, it destroys 70%. As many as five segments
of the cord become transformed into a fluid-filled cavity, which
is replaced with collagenous scar tissue over the next 3 to 4 weeks. This
scar is one of the obstacles to the regeneration of lost nerve fibers.
Treatment
The first priority in treating a spinal injury patient is to immobilize the spine to prevent further injury to the cord. Respiratory or other life support may also be required. Methylprednisolone, a steroid, dramatically improves recovery. Given within 3 hours of the trauma, it reduces injury to cell membranes and inhibits inflammation and apoptosis.
After these immediate requirements are met, reduction (repair) of the fracture is important. If a CT or MRI scan indicates spinal cord compression by the vertebral canal, a decompression laminectomy may be performed, in which the vertebral arch is removed from the affected region. CT and MRI have helped a great deal in recent decades for assessing vertebral and spinal cord damage, guiding surgical treatment, and improving recovery. Physical therapy is important for maintaining muscle and joint function as well as promoting the patient’s psychological recovery.
Treatment of spinal cord injuries is a lively area of medical research today. Some current interests are the use of antioxidants to reduce free radical damage, and the implantation of embryonic stem cells, which has produced significant (but not perfect) recovery from spinal cord lesions in rats.
Before You Go On
Answer the following questions to test your understanding of the preceding section:
9. Name five structural components of a typical somatic reflex arc. Which of these is absent from a monosynaptic arc?
10. State the function of each of the following in a muscle spindle: intrafusal fiber, annulospiral ending, and motor neuron.
11. Explain how nerve fibers in a tendon sense the degree of tension in a muscle.
12. Why must the withdrawal reflex, but not the stretch reflex, involve a polysynaptic reflex arc?
13. Explain why the crossed extensor reflex must accompany a withdrawal reflex of the leg.