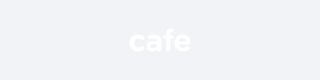
해부학, 생체역학, 인간의 움직임속에서 과부하걸린 조직의 이해, 그것을 바탕으로 이해한 pathology
그리고 정확한 진단
phyiscal exam
neurologic exam
x-ray, ct, mri
mri공부를 해야겠다. 진지하게
해부학적 구조와 Pathology에 대해서 완전하게 알지 않으면 MRI, 초음파 등의 진단도구를 이용할 수 없다.
panic bird..
Musculoskeletal MRI. pelvis and knee.pdf
INTRODUCTION
Imaging of the bones, joints, muscles, and cartilage represents the radiologic subspecialty of musculoskeletal imaging. Musculoskeletal imaging can be performed using a number of modalities, including magnetic resonance imaging (MRI), computed tomography (CT), ultrasonography, nuclear medicine, radiography, and fluoroscopy. Radiography is predominantly used for bony imaging due to the extremely high detail achievable with this technique and also because of its comparatively lower cost. However, bony injuries are often accompanied by injuries to the joint cartilage and muscles, tendons, and ligaments. A bone bruise, or contusion, is difficult or impossible to detect using radiography, even though radiography excels at demonstrating structural and anatomical detail such as fractures, tumors, or arthritic changes.
MRI is now the gold standard for most types of musculoskeletal imaging. The strength of MRI in imaging physiologic processes is due to its combined strengths of high detail, multiplanar imaging capability, lack of ionizing radiation, and sensitivity to physiologic changes. MRI also excels in the ability to distinguish various types of tissues as a result of their water and fat content. Because MRI provides a wide range of choices for imaging in the form of various pulse sequences and options, it allows for a very comprehensive evaluation of an injury in a fairly short period of time.
MRI OF SPECIFIC JOINTS AND EXTREMITIES
The scope of this article is to provide the technologist with realistic and easily applicable guidelines for MRI imaging of joints and extremities. Due to the complexity of many of these procedures and the number of joints, this installment will cover only the
Bony pelvis
Knee
Shoulder
Elbow
The Bony Pelvis
The bony pelvis is composed of the iliac bones, the sacrum and coccyx, the pubis and ischium, and the proximal femurs. These bony structures are connected by a very complex network of muscles and cartilaginous structures. This assembly provides a fairly rigid support structure that allows the upper body to be carried by the legs during ambulation and sitting. The bony pelvis also provides structural support and protection for the organs of the pelvis-the bladder, bowel, and organs of reproduction.
Anatomy of the Bony Pelvis
In addition to the bony structures and organs in the pelvis, there are numerous complex muscle groups. These include the muscles of the buttock (gluteus maximus, medius, and minimus); muscles of the anterior abdomen (rectus and transversus abdomimus, pyramidalis, and external and internal oblique muscles); muscles of the lower back (quadratus lumborum, latissimus dorsi, and iliopsoas muscles); muscles of the pelvis and pelvic floor (iliacus, quadratus femoris, pectineus, obturator internus and externus, levator ani, piriformis, and gemellus groups); and muscles of the superior thigh (adductor magnus, brevis, and longus; gracilis; sartorius; and quadriceps and hamstring groups [to be discussed in greater detail later]).
Pathology
Traumatic injury such as those suffered by athletes often affects the muscles, tendons, ligaments, or cartilage. Bony involvement is seen as well, but often manifests as bone contusion or marrow edema as a result of impact or abnormal movement, especially that related to hyperextension injuries. Evaluation of the acetabular labrum can be achieved using conventional MRI, but a more definitive diagnosis is often made using MR arthrography. In this setting it is important to include some high-resolution scans that are limited to the affected area to adequately image this smaller structure. ON occurs in the femoral heads, frequently bilaterally. This process is well documented using MRI. Although it does not require high resolution to make the diagnosis of ON, assessment of the degree of ON involvement along the articular surface of the femoral head does require high resolution. This can generally be achieved with a single additional series. Pain in the pelvic region can also be referred from soft tissue injuries or processes. What begins as a musculoskeletal examination can ultimately turn into a diagnosis of a soft-tissue tumor or a deep hematoma within the pelvic cavity. Circumstances like this are why we believe it is important not to limit the examination to only the region of the acetabula.
Techniques
For hip/pelvic imaging in the majority of our patients, a pelvic or torso surface array coil is used. For patients too large to be effectively accommodated with this type of coil, the integrated body coil is used.
Localizer-Three Plane
Gradient echo: Scan time < 60 sec
5-9 slices per plane
10.0-mm thickness with 5.0-mm gap
FOV (field-of-view) 480 mm
Matrix 256 F (frequency) x 128 P (phase)
T1-weighted spin echo (SE) or fast spin echo (FSE) (TE: 11-20 mm)
Axial plane
Whole-pelvis coverage
6.0- to 8.0-mm thickness with 1.0-mm gap
FOV to fit L-R dimension of pelvis (typically 320-440 mm)
Matrix 320 F x 192 P (512 interpolated)
Phase axis R-L
100% phase oversampling
Spatial presaturation slabs superior and inferior to slice volume
T2-weighted FSE (TE: 70-80 ms) with spectral fat suppression
Axial plane
Whole-pelvis coverage-matched to T1 axial
6.0- to 8.0-mm thickness with 1.0-mm gap
FOV to fit L-R dimension of pelvis (typically 320-440 mm)
Matrix 320 F x 192 P (512 interpolated)
Phase axis R-L
100% phase oversampling
Spatial presaturation slabs superior and inferior to slice volume
T1-weighted SE or FSE high resolution (TE: 11-20 ms)
Coronal plane
Coverage from posterior coccyx through anterior ubic symphysis
5.0- to 6.0-mm thickness with 1.0-mm gap
FOV to include lateral skin surfaces (typically 420-480 mm)
Matrix 512 F x 320 P (512 interpolated)
Phase oversampling at operator discretion
Fast STIR (FSE-IR) (TE: 45-55 ms)
Coronal plane
Inversion time (TI) is field strength-dependent: 130 ms at 1.5 T, 110 ms at 1.0 T, 90 ms at 0.7 T
Coverage from posterior coccyx through anterior pubic symphysis-matched to T1 coronal
5.0- to 6.0-mm thickness with 1.0-mm gap
FOV to include lateral skin surfaces (typically 420-480 mm)
Matrix 320 F x 192 P (512 interpolated)
Phase oversampling at operator discretion
Proton density (PD)-weighted (TE: 36 ms) FSE with spectral fat suppression
Coronal plane
Not scanned if ON seen on previous series-only for labral evaluation
Affected side(s) only-centered over acetabulum
Coverage through acetabulum with additional 10 mm anterior and posterior
4.0-to 5.0-mm thickness contiguous
FOV 200-220 mm
Matrix 256 F x 192 P (512 interpolated)
100% phase oversampling
Phase axis R-L
PD-weighted (TE: 18 ms) FSE
Sagittal plane
Only scanned if ON is seen or suspected
Affected side(s) only-centered over femoral head
Coverage through femoral head with additional 5 mm medial and lateral
4.0-to 5.0-mm thickness contiguous
FOV 200-220 mm
Matrix 256 F x 224 P (512 interpolated)
100% phase oversampling
Phase axis A-P
Additional sequences are occasionally added after the radiologist’s review, such as oblique thin section coronal and axial to evaluate the sacroiliac joints, or thin section axials for acetabular irregularities. Generally, the examination can be completed comfortably in a 30-minute time slot.
The Knee
The knee joint is a hinge joint and consists, in the broadest sense, of four bones articulating in different ways. Normal motion is flexion and extension. The distal femur articulates with both the proximal tibia and patella; the tibia articulates with the patella, distal femur, and proximal fibula.
Soft Tissues and Cartilage
Soft-tissue structures in the knee include the medial and lateral menisci, the anterior and posterior cruciate ligaments; medial (MCL) and fibular (FCL) collateral ligaments; patellar ligament and quadriceps tendon; patellar, tibial, and femoral articular cartilage; and the muscles of the thigh and calf. The articular surfaces of the femoral condyles, the tibial plateau, and the posterior patella are lined with a layer of hyaline cartilage. The muscles of the mid- and distal thigh involved in knee movement consist of the quadriceps group anteriorly (rectus femoris and vastus lateralis, intermedius, and medialis); the hamstring group posteriorly (long and short heads of the biceps femoris, semitendinosus, and semimembranosus); and the gracilis and sartorius muscles medially. The muscles of the proximal and mid-calf involved in knee movement include the gastrocnemius, popliteus, and peroneus longus muscles. There are also several fluid-filled sacs called bursae that act as cushions between tendons during movement.
Bony Structures
As previously mentioned, the knee joint is comprised of the femur, patella, tibia, and fibula. In some patients, a variant sesamoid bone called the fabella is also seen in the lateral gastrocnemius tendon. The primary articulations of the knee are between the posterior surfaces of the patella and the anterior/inferior surfaces of the femur, and the superior surfaces of the tibia and the inferior/posterior surfaces of the femur. The proximal tibio-fibular articulation is a fibrous articulation that does not permit very much movement.
Pathology
The most common traumatic injuries seen in the knee are tears of the menisci, tears of the anterior cruciate ligament (ACL), chondral injuries, tears of the medial collateral ligament (MCL), and transient patellar dislocations. Degenerative changes in the knee unrelated to trauma are also very common and include articular cartilage loss (chondromalacia), meniscal degeneration, and patellar cartilage degeneration as well as bony changes related to asymmetric weight bearing and repetitive motion injury.
Another frequently seen injury, particularly in athletes, is stress fracture. This can often involve the tibial plateau, but frequently extends distally along the metaphysis. In this setting, a complete evaluation for a stress fracture should include interrogation of the entire portion of the tibia if there is marrow edema extending beyond the field of view of the knee examination.
Baker’s cysts are another phenomenon seen on an MRI examination of the knee. These cystic structures commonly result from abnormally enlarged bursa between the medial head of the gastrocnemius muscle and semimembranosus tendons. On occasion, Baker’s cysts can rupture, causing the synovial fluid contained within to be released into the area surrounding the muscles. This can be quite painful because the fluid dissects along the intermuscular spaces and gives a MR appearance of high T2 signal intensity surrounding the muscles and tendons in the area of the ruptured cyst.
Abnormal accumulations of fluid in various compartments of the knee are frequently seen with traumatic injury to ligaments such as the ACL, PCL, and MCL as well as tears of the menisci or ruptures of the patellar ligament and quadriceps tendon. These fluid collections are generally indications of the injuries that produced them.
A wide variety of less common pathologies are also seen on an MR knee examination, including bony lesions such as benign and malignant neoplasms, osteonecrosis, bone cysts, bony changes resulting from reflex sympathetic dystrophy (RSD) and processes involving the soft tissues such as rhabdomyosarcoma, tenosynovitis, and a rare disorder called pigmented villonodular synovitis. Metabolic processes can also be visualized on MRI, including yellow-red marrow reconversion, osteopenia, and osteoporosis. In addition, aneurysms of the popliteal artery can be well visualized with MRI.
Common Mechanisms of Injury
Most traumatic injuries of the knee are related to atypical motion (twisting, lateral flexion, or extension) or exceeding the normal range of motion (hyperextension). These injuries are very common in sports such as basketball, football, soccer, and tennis and often result in cartilaginous and ligamentous injury. Impact injuries such as those sustained from a fall or an automobile accident, frequently result in fractures of the patella or tibial plateau. However, these two injury types are not mutually exclusive. With certain specific injuries such as ACL tears, there are often associated bony injuries in the form of contusions directly related to the mechanics of the motion that occurs as the ligament is torn. This can present a classic appearance on MRI in the form of a concomitant meniscal injury and bone bruise.
Technique
Our protocol for knee MRI consists of a localizer and five sequences. Care is taken when positioning the patient to ensure that the area of the menisci is centered to the center of the coil. We use a transmit-receive surface coil for imaging the knee.
Localizer-Three Plane
Gradient echo: Scan time < 60 sec
5-7 slices per plane
8.0-mm thickness with 2.0-mm gap
FOV 260 mm
Matrix 256 F x 128 P
PD-weighted (TE: 36 ms) FSE with spectral fat suppression
Axial plane
Coverage from above patella through superior tibial tuberosity
4.0-mm thickness with 0.4- to 1.0-mm gap
FOV 160 mm
Matrix 288 F x 192 P (512 interpolated)
Phase axis R-L
Spatial presaturation slabs superior and inferior to slice volume
This series allows for a sensitive evaluation of the patellar articular cartilage and retinacular structures and gives a secondary view of the ACL and menisci; the series also allows for evaluation of structures in the popliteal fossa.
PD-weighted (TE: 26 ms) FSE
Sagittal plane
Coverage from MCL medially through FCL laterally (inclusive)
Angulation is perpendicular to the plane intersecting the posterior margins of the femoral condyles
3.0-mm with 1.0-mm gap
FOV 160 mm
Matrix 320-384 F x 192-224 P (512 interpolated)
Phase axis S-I
100% phase oversampling
Spatial presaturation slabs superior and inferior to slice volume
This series allows for a comprehensive evaluation of the patellar ligament and quadriceps tendon, ACL and PCL, and also the menisci.
T2-weighted (TE: 115-120 ms) FSE
Sagittal plane
Coverage from MCL medially through FCL laterally (inclusive)
Matched to PD sagittal
Angulation is perpendicular to the plane intersecting the posterior margins of the femoral condyles
3.0-mm with 1.0-mm gap
FOV 160 mm
Matrix 320 F x 192 P (512 interpolated)
Phase axis S-I
100% phase oversampling
Spatial presaturation slabs superior and inferior to slice volume
This series allows for evaluation of the joint capsule and comparison of fluid and tissue intensities based on T2 values.
T2-weighted (TE: 70-80 ms) FSE with spectral fat suppression
Coronal plane
Coverage from popliteal artery posteriorly through patella anteriorly
Angulation is parallel to the plane intersecting the posterior margins of the femoral condyles
4.0-mm thickness with 1.0-mm gap
FOV 160 mm
Matrix 320 F x 192 P (512 interpolated)
Phase axis R-L
Spatial presaturation slabs superior and inferior to slice volume
We use this series to evaluate the bony structures for contusion and also to visualize the femoral and tibial articular cartilage and evaluate for subchondral injury. It also provides another second look at the ACL and menisci.
T1-weighted SE (TE: 17 ms) high resolution
Coronal plane
Coverage from popliteal artery posteriorly through patella anteriorly
Angulation is parallel to a line intersecting posterior margins of femoral condyles
4.0-mm thickness with 1.0-mm gap
FOV 160 mm
Matrix 448 F x 224 P (512 interpolated)
Phase axis R-L
This series gives us a good means of evaluating the menisci, bony structures, articular surfaces, MCL, and FCL.
The Shoulder
The shoulder is a complex joint in that it is the sole attachment of the upper extremity to the torso. The articulation between the humeral head and the glenoid process of the scapula is the joint responsible for abduction, adduction, and rotation of the upper extremity. The acromial extremity of the clavicle articulates with the acromion process of the scapula to form a fibrous joint known as the acromioclavicular (AC) joint.
Soft Tissues, Muscles, and Cartilage
The cartilaginous, ligamentous, and myotendinous structures of the shoulder interact in a complex manner to allow for the range and variety of movements possible at the shoulder. The glenohumeral joint itself is a ball-and-socket joint. The rim of the glenoid fossa is lined with a cartilaginous lip known as the glenoid labrum. The rotational motion of the humerus is a result of the complex interaction of four muscles-the supraspinatus, infraspinatus, subscapularis and teres minor-that together form the tendons of the rotator cuff. Other muscles that participate in movement of the shoulder include the deltoid group, pectoralis major, latissimus dorsi, biceps brachii, triceps brachii, teres major, and coracobrachialis.
Bony Structures
The bones of the shoulder girdle are the scapula, humerus, and clavicle. The primary mobile articulation is at the glenoid fossa involving the scapula and the humeral head.
Pathology
Due to the range and complexity of motion in the shoulder and the stresses it is subjected to, traumatic injuries to both the bony and soft tissue structures of this area are common. The glenoid labrum is a frequent site of injury due to the inherent instability of the articulation between the humeral head and the relatively shallow glenoid fossa. Dislocations commonly result. Cartilaginous tears occur in many classic patterns. SLAP lesions (superior labrum anterior to posterior), which occur at the insertion of the biceps tendon into the labrum, and Bankart lesions, which occur at the attachment of the inferior glenohumeral ligament to the labrum anteroinferiorly, are among the more common. Included in the category of Bankart lesions are Perthes lesions and ALPSA (anterior labroligamentous periosteal sleeve avulsions).
Another type of injury involving the articular surface of the glenoid labral cartilage rather than the rim is a GLAD lesion (glenohumeral articular disruption). Labral and articular surface tears are very common sequelae of shoulder dislocations. Additionally, dislocations—either acute and isolated, or chronic—can result in indentations or grooves in the articular surface of the humeral head. Specifically in the case of anterior dislocations, these lesions are termed Hill-Sachs lesions or deformities.
Tears of the rotator cuff tendons are also among the more common injuries seen, particularly among athletes. Rotator cuff tears can involve any single tendon of the cuff or a combination of the four tendons; these tears can be partial or complete and often involve the inferior surface of the tendons (as in partial undersurface tears). Tears and tendinopathy of the rotator cuff tendons are often the result of impingement (rubbing or squeezing of the tendon(s) against or between adjacent bony structures) and are often—but not always—accompanied by inflammation of bursae (bursitis).
Fractures of the glenoid and humeral head are also seen, and the frequency increases with the degree of trauma. In elderly and osteoporotic patients, humeral head fractures are often seen and can be severe enough to require prosthetic replacement. Separation of the fibrous joint between the distal clavicle and scapular acromion are also frequently seen as a result of trauma. Non-traumatic abnormalities include osteonecrosis, neoplastic lesions (either primary or metastatic) and marrow reconversion.
Common Mechanisms of Injury
The most common shoulder injuries are a result of either a chronic, repetitive nature (impingement) or an acute, traumatic nature (instability and dislocation or fracture). These injuries are very frequently seen in athletes participating in sports that require significant shoulder use, such as baseball, tennis, football, swimming, weight lifting, rowing, and track and field events (e.g., shot put, javelin throw).
Technique
Our approach to shoulder imaging is comprehensive while only requiring four sequences as routine. We use a four-channel unilateral phased array coil centered over the affected shoulder. The patient is ideally positioned with the arm next to the body (adducted) and in as much external rotation as the patient will tolerate.
Localizer-Three Plane
Gradient echo: Scan time < 60 sec
5-7 slices per plane
8.0-mm thickness with 2.0-mm gap
FOV 260 mm
Matrix 256 F x 128 P
PD-weighted (TE: 36 ms) FSE with spectral fat suppression
Axial plane
Coverage from AC joint superiorly to 10 mm below inferior margin of glenoid labrum
4.0-mm thickness with 1.0-mm gap
FOV 160 mm
Matrix 256 F x 256 P (512 interpolated)
100% phase oversampling
Phase axis A-P
This series provides us with good visualization of the anterior and posterior labrum, as well as the muscles of the shoulder and the humeral head
T1-weighted (TE: 11-20 ms) SE or FSE
Oblique coronal plane
Coverage 10 mm posterior-lateral to humeral head through 10 mm anteromedial to humeral head
Angulation parallel to plane of supraspinatus tendon
3.0- to 3.5-mm thickness with 0.5- to 1.0-mm gap
FOV 140-160 mm
Matrix 448 F x 224 P (512 interpolated)
100% phase oversampling
Phase axis A-P
This series provides excellent visualization of the superior and inferior glenoid labrum and articular cartilage of both the glenoid fossa and humeral head.
T2-weighted (TE: 70-80 ms) FSE with spectral fat suppression
Oblique coronal plane
Coverage 10 mm posterior-lateral to humeral head through 10 mm anteromedial to humeral head
Angulation parallel to plane of supraspinatus tendon
3.0- to 3.5-mm thickness with 0.5- to 1.0-mm gap
FOV 140-160 mm
Matrix 320 F x 192 P (512 interpolated)
100% phase oversampling
Phase axis A-P
This series provides excellent visualization of the rotator cuff tendons and is sensitive to acute bony changes resulting from trauma, as well as providing visualization of the superior and inferior glenoid labrum.
T2-weighted (TE: 70-80 ms) FSE with spectral fat suppression
Oblique sagittal plane
Coverage from neck of glenoid posteromedially into deltoid muscle anterolaterally
Angulation perpendicular to plane of supraspinatus tendon
3.5- to 4.0-mm thickness with 1.0-mm gap
FOV 140-160 mm
Matrix 320 F x 192 P (512 interpolated)
100% phase oversampling
Phase axis A-P
This series provides exceptional cross-sectional evaluation of the rotator cuff tendons, the biceps tendon, the subacromial subdeltoid bursa, and the superior surface of the humeral head.
Fast STIR (FSE-IR) (TE: 45-55 ms) optional
Used as a substitute for T2 with spectral Fat Sat if off-isocenter fat suppression is unreliable
Oblique coronal or oblique sagittal plane
TI is field strength-dependent: 130 ms at 1.5 T, 110 ms at 1.0 T, 90 ms at 0.7 T
Other parameters identical to T2 fat suppressed series
If the supraspinatus tendon is not well visualized on the initial localizer, an additional axial localizer is performed using either a gradient echo sequence or a PD-weighted FSE sequence. Either of these scans is less than 90 seconds in duration. For the specific evaluation of labral injuries, we often perform MR arthrography. In that setting, we acquire two planes of T1-weighted FSE with spectral Fat Sat (oblique coronal and oblique sagittal), axial T1-weighted FSE without Fat Sat, and an oblique coronal T2-weighted series with spectral Fat Sat. These scans are all performed with the arm in adduction and external rotation. To facilitate evaluation of the inferior glenohumeral ligament, we reposition the patient with the arm in abduction and external rotation (ABER) and (after a localizer) acquire oblique sagittal spectral fat-suppressed T1-weighted FSE scans. The slices are planned off of a coronal slice through the mid-glenohumeral articulation and are aligned with the long axis of the humeral metaphysis. Due to the position of the patient’s arm for this scan, it is often not possible to use the array coil. In this case, we use either a linear flex coil or a 5-inch circular surface coil.
The Elbow
The elbow is a combination hinge and condyloid joint. The motion of the elbow includes supination and pronation (of the forearm) as well as flexion and extension of the elbow joint. The flexion-extension is a result of the ulnohumeral articulation (hinge) and the supination-pronation is a result of the radiohumeral articulation (condyloid). As with the knee, hyperextension injuries are not uncommon, but because the elbow is not a weight-bearing joint, twist injuries are less common.
Soft Tissue, Muscles, and Cartilage
Muscles involved with elbow movement include the biceps brachii, triceps brachii, brachialis, brachioradialis, anconeus, pronator teres, and supinator muscles. Articular cartilage lines the articulating surfaces of the radial head, ulnar olecranon and trochlea, and capitellum of the humerus. The radial collateral and ulnar collateral ligaments provide stability to the lateral and medial sides of the joint, respectively.
Bony Structures
The bones that form the elbow joint are the distal humerus and the proximal radius and ulna. The olecranon process of the ulna articulates with the trochlear portion of the humerus medially. The radial head articulates with the capitellar portion of the humerus laterally. Additionally, the radial head and the radial notch of the ulna articulate during supination and pronation motion of the forearm.
Pathology
The most common traumatic injuries of the elbow are ruptures of the biceps tendon, tears of the ulnar collateral ligament, and fractures of the radial head and humeral epicondyles. Overuse or repetitive use injuries, such as tennis elbow and extensor tendinopathy are common. Flexor tendinopathy is often seen in unskilled golfers due to the motion associated with forceful swinging of the golf club and hitting the ground, resulting in sudden deceleration. Also, masses in the cubital tunnel can cause compression of the ulnar nerve that result in pain and paresthesias distally.
Common Mechanisms of Injury
The most common mechanisms of injury to the elbow are hyperextension injuries, repetitive motion injuries, rapid deceleration injuries, resistance- related injuries, and so-called valgus stress injuries. Hyperextension injuries most frequently result in biceps tendon tears but can also be associated with fractures of the olecranon and radial head, depending on the degree of hyperextension. Repetitive motion injuries are common in sports where specific motions under force are repeated.
Classic examples of this are racquet sports, golf, and baseball; in the specific case of baseball pitchers, overhand pitching commonly results in the aforementioned valgus stress injury. Resistance- related injuries are frequent with weightlifting and often manifest in the form of tendon ruptures and tears due to the contraction of the muscle under resistance. Injuries to tendons are also associated with courses of systemic antibiotic therapy, particularly with fluoroquinolone antibiotics such as ciprofloxacin, which can cause transient changes in the tendons that result in structural weakening. Although fluoroquinolone-related tendinous injuries most frequently occur with the Achilles tendon, they are possible anywhere in the body, especially with muscles under resistance.
Technique
Our preference is to use a transmit-receive surface coil or multichannel surface array such as a knee/extremity coil for elbow imaging. This assumes that the patient can extend his or her arm above the head from the shoulder and maintain that position for roughly the 15 minutes required for imaging. Patients unable to assume and maintain this position are imaged on our open MRI. We have found that far off-isocenter imaging of the elbow on our high-field cylindrical magnets does not provide satisfactory results. For this reason, imaging the elbow with the patient’s arm by the side is only used as a last resort. Ideally, we try to have the patient positioned with the hand supinated relative to the antecubitus. Care is taken to assure that we have localizers that allow us to align our sagittal and coronal series along the long axes of the humerus and radius/ulna.
Localizer-Three Plane
Gradient echo: Scan time < 60 sec
5-7 slices per plane
8.0-mm thickness with 2.0-mm gap
FOV 260 mm
Matrix 256 F x 128 P
PD-weighted (TE: 30 ms) FSE
Axial plane
Coverage from 15-20 mm proximal to humeral epicondyles to 15 mm distal to radial tuberosity
4.0-mm thickness with 1.0-mm gap
FOV 140 mm
Matrix 288 F x 192 P (512 interpolated)
Spatial presaturation slabs superior and inferior to slice volume
Phase axis A-P
T2-weighted (TE: 117 ms) FSE
Axial plane
Coverage from 15-20 mm proximal to humeral epicondyles to 15 mm distal to radial tuberosity
4.0-mm thickness with 1.0-mm gap
FOV 140 mm
Matrix 288 F x 192 P (512 interpolated)
Spatial presaturation slabs superior and inferior to slice volume
Phase axis A-P
T1-weighted (TE: 13-20 ms) FSE
Oblique coronal plane
Coverage from posterior to triceps tendon insertion to 15 to 20 mm anterior to radial tuberosity
Angulation parallel to plane intersecting medial and lateral humeral epicondyles
3.0-mm thickness with 1.0-mm gap
FOV 140 mm
Matrix 320 F x 224 P (512 interpolated)
Phase axis R-L
T2-weighted (TE: 70-80 ms) FSE with spectral fat suppression
Oblique coronal plane
Coverage from posterior to triceps tendon insertion to 15 to 20 mm anterior to radial tuberosity
Angulation parallel to plane intersecting medial and lateral humeral epicondyles
3.0-mm thickness with 1.0-mm gap
FOV 140 mm
Matrix 256 F x 192 P (512 interpolated)
Phase axis R-L
Spatial presaturation slabs superior and inferior to slice volume
T2-weighted (TE: 117 ms) FSE
Oblique sagittal plane
Coverage from 12 mm medial to medial humeral epicondyle to 12 mm lateral to lateral humeral epicondyle
Angulation perpendicular to plane intersecting medial and lateral humeral epicondyles
4.0-mm thickness with 1.0-mm gap
FOV 140 mm
Matrix 320 F x 192 P (512 interpolated)
Spatial presaturation slabs superior and inferior to slice volume
Phase axis A-P
T2*-weighted gradient echo (TE: 20 ms/Flip angle 15 degrees) optional
Used for visualization of articular cartilage
Oblique coronal plane
Coverage from triceps tendon insertion to radial tuberosity
Angulation parallel to plane intersecting medial and lateral humeral epicondyles
3.0-mm thickness contiguous
FOV 140 mm
Matrix 288 F x 192 P
Phase axis R-L
SUMMARY
Musculoskeletal imaging can be accomplished with a number of different modalities, including radiography, fluoroscopic arthrography, sonography, nuclear medicine scinitigraphy, CT, and MRI. Musculoskeletal imaging is unequivocally one of the core competencies of MRI due to the flexibility of imaging planes and sequences and the high spatial detail possible. Musculoskeletal MRI is not limited solely to the imaging of sports-related injuries. MRI excels at depicting any processes—whether traumatic, neoplastic, or metabolic—that affect the bones or soft tissues of the body. MRI is also not limited to imaging only the joints. MRI is highly sensitive to injuries and processes involving the bones and muscles of the extremities themselves and can be made even more sensitive and specific with respect to inflammatory and neoplastic processes through the use of gadolinium contrast agents.