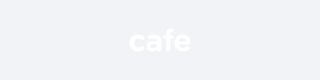
Oxid Med Cell Longev. 2019; 2019: 9426867.
Published online 2019 Feb 20. doi: 10.1155/2019/9426867
PMCID: PMC6402202
PMID: 30915197
Benefits of Vitamins in the Treatment of Parkinson's Disease
Xiuzhen Zhao, 1 Ming Zhang, 2 Chunxiao Li, 3 Xue Jiang, 1 Yana Su, 1 and Ying Zhang
1
Author information Article notes Copyright and License information PMC Disclaimer
Abstract
Parkinson's disease (PD) is the second most common neurodegenerative disease in the elderly, which is clinically characterized by bradykinesia, resting tremor, abnormal posture balance, and hypermyotonia. Currently, the pathogenic mechanism of PD remains unclear. Numerous clinical studies as well as animal and cell experiments have found a certain relationship between the vitamin family and PD. The antioxidant properties of vitamins and their biological functions of regulating gene expression may be beneficial for the treatment of PD. Current clinical evidence indicates that proper supplementation of various vitamins can reduce the incidence of PD in the general population and improve the clinical symptoms of patients with PD; nevertheless, the safety of regular vitamin supplements still needs to be highlighted. Vitamin supplementation may be an effective adjuvant treatment for PD. In this review, we summarized the biological correlations between vitamins and PD as well as the underlying pathophysiological mechanisms. Additionally, we elaborated the therapeutic potentials of vitamins for PD.
파킨슨병(PD)은 노인에서 두 번째로 흔한 신경 퇴행성 질환으로, 임상적으로 서동증, 안정 시 진전, 자세 균형 이상, 근긴장 이상증이 특징입니다. 현재 파킨슨병의 발병 기전은 아직 명확하지 않습니다. 수많은 임상 연구와 동물 및 세포 실험을 통해 비타민 계열과 PD 사이의 특정 관계가 밝혀졌습니다.
비타민의 항산화 특성과
유전자 발현을 조절하는 비타민의 생물학적 기능은
PD 치료에 도움이 될 수 있습니다.
현재 임상 증거에 따르면 다양한 비타민을 적절히 보충하면
일반 인구의 PD 발병률을 낮추고
PD 환자의 임상 증상을 개선할 수 있지만,
일반 비타민 보충제의 안전성은 여전히 강조되어야 합니다.
비타민 보충제는
파킨슨 병에 효과적인 보조 치료제가 될 수 있습니다.
이 리뷰에서는 비타민과 PD 사이의 생물학적 상관관계와 근본적인 병태생리학적 메커니즘을 요약했습니다. 또한 PD에 대한 비타민의 치료 잠재력을 자세히 설명했습니다.
1. Introduction
Parkinson's disease (PD) is the second most common neurodegenerative disorder following Alzheimer's disease. Clinically, PD is characterized by resting tremor, hypermyotonia, postural instability, and bradykinesia [1]. Additionally, patients with PD can also manifest with nonmotor symptoms, such as cognitive decline, olfactory dysfunction, constipation, sleep disorders, and autonomic symptoms [2], and these nonmotor symptoms usually occur prior to the onset of motor symptoms [3]. PD severely affects the quality of life of the individual with the disease and also creates a great burden on the caregivers. The typical pathological hallmark of PD is degeneration of dopaminergic neurons in the substantia nigra pars compacta (SNpc) and eosinophilic inclusion bodies (Lewy bodies) in the remaining neurons, which is the major contributor to the deficiency of dopamine in the basal ganglia [4, 5]. The exact pathogenetic mechanisms of PD is not yet fully understood. Current theories regard PD as a multifactorial disease, involving various genetic and environmental factors, among which mitochondrial dysfunction and oxidative stress play an important role in the pathogenesis and development of PD [6, 7]. The treatment for PD is challenging, and the existing therapeutic strategies can only relieve clinical symptoms but fail to control the progression of PD.
Vitamins are natural bioactive products with antioxidant properties, which are necessities for maintaining the normal functions of human organisms. Essential vitamins cannot be endogenously synthesized in the organism and therefore must be obtained through the diet. Clinically, vitamin deficiency is quite common, especially in infants and elderly. Vitamins are generally divided into fat-soluble variants (vitamins A, D, E, and K) and water-soluble variants (vitamins B and C). The former mainly bind to cellular nuclear receptors and affect the expression of specific genes [8]. The latter mainly constitute a cofactor for the enzyme, affecting the enzymatic activity [9].
Numerous clinical studies as well as animal and cell experiments have found a certain relationship between the vitamin family and PD [10]. The antioxidant properties of vitamins and their biological functions of regulating gene expression may be beneficial for the treatment of PD. Current clinical evidence indicates that proper supplementation of various vitamins can reduce the incidence of PD in the general population and improve the clinical symptoms of patients with PD; nevertheless, the safety of regular vitamin supplements still needs to be highlighted. Vitamin supplementation may represent an effective adjuvant treatment for PD. In this review, we summarized the biological correlations between vitamins and PD as well as the underlying pathophysiological mechanisms. Additionally, we elaborated the therapeutic potentials of vitamins for PD.
1. 소개
파킨슨병(PD)은
알츠하이머병에 이어 두 번째로 흔한 신경 퇴행성 질환입니다.
임상적으로 PD는 안정 시 진전, 근긴장 이상, 자세 불안정성, 서동 운동증이 특징입니다[1]. 또한 PD 환자는 인지 기능 저하, 후각 기능 장애, 변비, 수면 장애, 자율 신경 증상과 같은 비운동 증상도 나타날 수 있으며[2], 이러한 비운동 증상은 일반적으로 운동 증상이 시작되기 전에 발생합니다[3]. 파킨슨병은 환자의 삶의 질에 심각한 영향을 미치며 간병인에게도 큰 부담을 줍니다.
PD의 전형적인 병리학적 특징은
흑질 흑질(SNpc)의 도파민 신경세포의 퇴화와
나머지 신경세포의 호산구 봉입체(Lewy body)로,
이는 기저핵의 도파민 결핍의 주요 원인입니다 [4, 5].
PD의 정확한 발병 기전은 아직 완전히 밝혀지지 않았습니다. 현재 이론에 따르면 PD는 다양한 유전적 및 환경적 요인이 관여하는 다인성 질환으로 간주되며, 그중 미토콘드리아 기능 장애와 산화 스트레스가 PD의 발병과 발달에 중요한 역할을 합니다 [6, 7]. 파킨슨병의 치료는 까다롭고 기존의 치료 전략은 임상 증상을 완화할 뿐 파킨슨병의 진행을 제어하지 못합니다.
비타민은
항산화 특성을 가진 천연 생리 활성 제품으로
인체 유기체의 정상적인 기능 유지에 필수적인 물질입니다.
필수 비타민은
유기체에서 내인성 합성이 불가능하므로
식단을 통해 섭취해야 합니다.
임상적으로 비타민 결핍은
특히 영유아와 노인에게 매우 흔합니다.
비타민은 일반적으로
지용성 변이체(비타민 A, D, E, K)와
수용성 변이체(비타민 B, C)로 나뉩니다.
전자(지용성 비타민)는
주로 세포 핵 수용체에 결합하여
특정 유전자의 발현에 영향을 미칩니다[8].
후자는
주로 효소의 보조 인자를 구성하여
효소 활성에 영향을 미칩니다 [9].
수많은 임상 연구와 동물 및 세포 실험에서 비타민 계열과 PD 사이의 특정 관계가 발견되었습니다 [10]. 비타민의 항산화 특성과 유전자 발현을 조절하는 생물학적 기능은 PD 치료에 도움이 될 수 있습니다. 현재 임상 증거에 따르면 다양한 비타민을 적절히 보충하면 일반 인구의 PD 발병률을 낮추고 PD 환자의 임상 증상을 개선할 수 있지만, 일반 비타민 보충제의 안전성은 여전히 강조될 필요가 있습니다. 비타민 보충제는 PD의 효과적인 보조 치료법이 될 수 있습니다. 이 리뷰에서는 비타민과 PD 사이의 생물학적 상관관계와 근본적인 병태생리학적 메커니즘을 요약했습니다. 또한 PD에 대한 비타민의 치료 잠재력을 자세히 설명했습니다.
2. The Pathogenesis of Oxidative Stress in PD
Oxidative stress refers to the imbalance between the oxidation system and antioxidant system, resulting in excessive accumulation of oxidative substances, such as reactive oxygen species (ROS) and reactive nitrogen species (RNS) [11]. ROS include superoxide anion radical (O2−), hydroxyl radical (OH−), and hydrogen peroxide (H2O2); RNS include nitric oxide (NO), nitrogen dioxide (NO2), and peroxynitrite (ONOO−). The antioxidant system mainly consists of two subtypes: (1) enzymatic antioxidant system, including superoxide dismutase (SOD), catalase (CAT), and glutathione peroxidase (GSH-Px), and (2) nonenzymatic antioxidant system, including vitamin C, vitamin E, glutathione, melatonin, alpha-lipoic acid, carotenoids, and trace elements copper, zinc, and selenium.
Oxidative stress plays an important physiological role in the organism. For example, phagocytic cells kill pathogenic microorganisms, participate in detoxification and enzymatic reactions, and synthesize some essential biologically active substances. Meanwhile, it can as well cause damage to the body, such as cell membrane destruction, protein denaturation, and nucleic acid changes.
There is increasing evidence that oxidative stress represents a pathophysiological characteristic of PD, and the production of reactive oxygen species can result in neuronal death [12, 13]. The mitochondrial respiratory chain is regarded as the major source of ROS [14]. Additionally, previous studies have found that mitochondrial dysfunction exists in the substantia nigra of patients with PD [15]. Reduced glutathione (GSH) can enhance the production of ROS and RNS [16], and oxidation of dopamine and dopamine metabolites such as 3,4-dihydroxyphenylacetic acid (DOPAC) can inhibit the activity of complex I [17]. These findings indicate that the downstream metabolites of dopamine may make dopamine neurons more susceptible. Moreover, iron accumulation in the substantia nigra is common in patients with PD, leading to overproduction of hydrogen peroxide and molecular oxygen in the Fenton reaction from Fe2+ to Fe3+; hydrogen peroxide generates a highly toxic hydroxyl radical through the Haber-Weiss reaction in the presence of Fe2+, which causes severe oxidative damage to the cellular components [18]. From the above, the oxidant stress is closely associated with the pathogenesis of PD. Oxidative stress can cause neuronal loss through some underlying intracellular damage, such as protein aggregation, mitochondrial dysfunction, and DNA rupture. Therefore, antioxidant damage has become a potential target for the treatment of PD.
2. PD에서 산화 스트레스의 발병 기전
산화 스트레스는
산화 시스템과 항산화 시스템 간의 불균형으로 인해
활성 산소 종(ROS) 및 반응성 질소 종(RNS)과 같은
산화 물질이 과도하게 축적되는 것을 말합니다[11].
ROS에는
슈퍼옥사이드 음이온 라디칼(O2-),
하이드록실 라디칼(OH-),
과산화수소(H2O2)가 포함되며,
RNS에는
산화질소(NO),
이산화질소(NO2),
과산화아질산염(ONOO-)이 포함됩니다.
항산화 시스템은 주로
(1) 슈퍼옥사이드 디스뮤타제(SOD), 카탈라아제(CAT), 글루타치온 퍼옥시다제(GSH-Px)를 포함한
효소 항산화 시스템과
(2) 비타민 C, 비타민 E, 글루타치온, 멜라토닌, 알파 리포산, 카로티노이드 및 미량 원소 구리, 아연, 셀레늄을 포함한
비효소 항산화 시스템의 두 가지 하위 유형으로 구성됩니다.
산화 스트레스는
유기체에서 중요한 생리적 역할을 합니다.
https://www.ncbi.nlm.nih.gov/pmc/articles/PMC4055301/
예를 들어,
식세포는 병원성 미생물을 죽이고
해독 및 효소 반응에 참여하며
필수적인 생물학적 활성 물질을 합성합니다.
한편
세포막 파괴,
단백질 변성,
핵산 변화 등 신체에 손상을 입힐 수도 있습니다.
산화 스트레스가
PD의 병리 생리학적 특성을 나타내며
활성 산소 종의 생성은
신경 세포 사멸을 초래할 수 있다는 증거가 증가하고 있습니다 [12, 13].
미토콘드리아 호흡 사슬은
ROS의 주요 원천으로 간주됩니다 [14].
또한, 이전 연구에 따르면
PD 환자의 흑질에
미토콘드리아 기능 장애가 존재한다는 사실이 밝혀졌습니다 [15].
감소된 글루타치온(GSH)은
ROS와 RNS의 생성을 촉진할 수 있으며[16],
도파민과 3,4-디하이드록시페닐아세트산(DOPAC)과 같은
도파민 대사 산물의 산화는
복합체 I의 활성을 억제할 수 있습니다[17].
이러한 연구 결과는
도파민의 하류 대사 산물이
도파민 뉴런을 더 취약하게 만들 수 있음을 나타냅니다.
또한,
흑질에 철이 축적되는 것은
PD 환자에서 흔하며,
Fe2+에서 Fe3+로의 펜톤 반응에서
과산화수소와 분자 산소의 과잉 생산으로 이어지고,
과산화수소는
Fe2+의 존재하에 하버-바이스 반응을 통해
독성이 강한 하이드 록실 라디칼을 생성하여
세포 성분에 심각한 산화적 손상을 일으킵니다 [18].
https://www.ncbi.nlm.nih.gov/pmc/articles/PMC10891198/
https://www.nature.com/articles/s41531-016-0004-y
위에서 살펴본 바와 같이
산화 스트레스는
PD의 발병과 밀접한 관련이 있습니다.
산화 스트레스는
단백질 응집,
미토콘드리아 기능 장애 및
DNA 파열과 같은
일부 근본적인 세포 내 손상을 통해
신경 세포 손실을 유발할 수 있습니다.
따라서
항산화 손상은
파킨슨병 치료의 잠재적 표적이 되었습니다.
3. Vitamin B and PD
The B family of vitamins is water-soluble, which includes thiamine (vitamin B1), riboflavin (vitamin B2), niacin (vitamin B3), pantothenic acid (vitamin B5), pyridoxine (vitamin B6), biotin (vitamin B7), folate (vitamin B9), and cobalamin (vitamin B12) [19]. These vitamins play an important role as enzyme cofactors in multiple biochemical pathways in all tissues, such as regulating metabolism, improving the function of the immune system and nervous system, and promoting cell growth and division [20].
Almost all of these B family vitamins are essential variants dependent on diet supply, except niacin which can also be synthesized from tryptophan. Vitamin B deficiencies are frequent in the children, elderly, vegetarians, pregnant women, and patients with gastrointestinal diseases. Recently, the association with vitamin B and PD is getting more and more attention. Herein, we use vitamin B3 as a representative to discuss the relationship between vitamin B and PD.
3. 비타민 B와 PD
비타민 B군은
티아민(비타민 B1),
리보플라빈(비타민 B2),
니아신(비타민 B3),
판토텐산(비타민 B5),
피리독신(비타민 B6),
비오틴(비타민 B7),
엽산(비타민 B9),
코발라민(비타민 B12)을 포함하는
수용성 비타민입니다[19].
이러한 비타민은
신진대사 조절,
면역계 및 신경계 기능 개선,
세포 성장 및 분열 촉진 등
모든 조직의 여러 생화학 경로에서 효소 보조 인자로서 중요한 역할을 합니다[20].
트립토판으로도 합성할 수 있는 니아신을 제외한
거의 모든 비타민 B군은
식단 공급에 의존하는 필수 비타민입니다.
비타민 B 결핍은
어린이, 노인, 채식주의자, 임산부 및 위장 질환 환자에게서
자주 발생합니다.
최근에는
비타민 B와 PD와의 연관성이 점점 더 주목받고 있습니다.
여기서는
비타민 B3를 대표적으로 사용하여
비타민 B와 PD의 관계를 논의합니다.
3.1. Vitamin B3
Nicotinamide is the active form of niacin, and it is the precursor of coenzymes NADH and NADPH, which are essential for over 200 enzymatic reactions in the organism, especially the production of adenosine triphosphate (ATP). Meat, fish, and wheat are generally rich in nicotinamide, while vegetables have a low nicotinamide content [21]. Deficiency of nicotinamide/niacin can lead to pellagra, causing dermatitis, diarrhea, and depression [22]. Nicotinamide has neuroprotective and antioxidant functions at low doses but exhibits neurotoxicity, especially dopaminergic toxicity, at high doses [23]. Fukushima also suggests that excessive nicotinamide is related to the development of PD [24]; excessive nicotinamide can induce overproduction of 1-methylnicotinamide (MNA), which is increased in patients with PD [25]. In an in vitro study, Griffin et al. found that low-dose nicotinamide (10 mM) has a significant effect on inducing differentiation from embryonic stem cells into neurons; however, higher doses (>20 mM) of nicotinamide induce cytotoxicity and cell death [26]. The definitive protective dose of vitamin B3 still needs further researches.
3.1. 비타민 B3
니코틴아마이드는
니아신의 활성 형태이며,
생체 내 200가지 이상의 효소 반응,
특히 아데노신 삼인산(ATP) 생성에 필수적인
코엔자임 NADH와 NADPH의 전구체입니다.
일반적으로
육류, 생선, 밀에는
니코틴아미드가 풍부하지만
채소에는 니코틴아미드 함량이 낮습니다[21].
니코틴아마이드/니아신이 결핍되면
피부염, 설사, 우울증을 유발하는
펠라그라가 발생할 수 있습니다 [22].
니코틴아마이드는 저용량에서는
신경 보호 및 항산화 기능이 있지만
고용량에서는 신경 독성,
특히 도파민 독성을 나타냅니다 [23].
후쿠시마는 또한
과도한 니코틴아마이드가
PD의 발병과 관련이 있다고 제안합니다 [24];
과도한 니코틴아마이드는
1-메틸니코틴아마이드(MNA)의 과생산을 유도할 수 있으며,
이는 PD 환자에서 증가합니다 [25].
시험관 내 연구에서 그리핀 등은 저용량 니코틴아마이드(10mM)가 배아 줄기세포에서 신경세포로의 분화를 유도하는 데 상당한 효과가 있지만 고용량(>20mM)의 니코틴아마이드는 세포 독성 및 세포 사멸을 유도한다는 사실을 발견했습니다[26]. 비타민 B3의 결정적인 보호 용량은 아직 추가 연구가 필요합니다.
3.2. Possible Neuroprotective Mechanisms of Vitamin B3 in PD
Firstly, numerous studies have demonstrated that mitochondrial dysfunction and cellular energy failure are pathophysiological features of PD. Nicotinamide participates in the biosynthesis of nicotinamide adenine dinucleotide (NAD; oxidized form: NAD+; reduced form: NADH) via various metabolic pathways [27]. NADH is an essential cofactor assisting the tetrahydrobiopterin functioning in tyrosine hydroxylase, which can hydroxylate tyrosine and produce dopamine; NADH deficiency is common in PD [28].
Secondly, NADH is indispensable for the physiological function of mitochondrial complex I in ATP synthesis, and the corresponding dysfunction is involved in PD patients and animal models [15, 29, 30]. Nicotinamide mononucleotide (NMN) constitutes one of the key precursors of NAD+. In previous in vitro studies, the scholars have established a cellular model of PD using rotenone-treated PC12 cells, and they found the NMN (0.1 mM or 1 mM) treatment was associated with a significantly higher survival rate in the rotenone-treated (0.5 μm) PC12 cells. NMN is assumed to enhance the intracellular levels of NAD+ and ATP in the cellular model of PD [31].
In addition, nicotinamide can act a neuroprotective role by inhibiting the oxidative stress. In 1-methyl-4-phenyl-1,2,3,6-tetrahydropyridine- (MPTP-) induced mouse models of PD, nicotinamide (500 mg/kg) was injected before subacute (30 mg/kg/d for 5 days) MPTP administration. This study showed that cotreatment with MPTP and nicotinamide significantly improved the locomotor activity compared to single-agent treatment with MPTP. Nicotinamide administration significantly attenuated the MPTP-induced dopamine depletion (47.11 ± 21.06 vs. 12.77 ± 8.06). Meanwhile, nicotinamide pretreatment markedly inhibited MPTP-induced lactate dehydrogenase (LDH) and NOS activities, which prevented the oxidative stress and alleviated the oxidative damage.
Sirtuins (SIRTs) are NAD+-dependent protein deacetylases involved in vital biological processes [32]. Recently, sirtuin 5 (SIRT5) has received considerable attention. Liu et al. investigated the role of SIRT5 in MPTP-induced mouse PD models [33]. They found that deletion of SIRT5 exacerbated motor deficits, nigrostriatal dopaminergic degeneration in the compact part of substantia nigra (SNc), and mitochondrial antioxidant activities in the PD models. These findings provide new insight into the therapeutic strategies for PD. However, the protective effects of nicotinamide are still controversial, and further researches are needed to clarify the biological function of vitamin B3 in PD.
3.2. 파킨슨병에서 비타민 B3의 가능한 신경 보호 메커니즘
첫째, 수많은 연구를 통해 미토콘드리아 기능 장애와
세포 에너지 장애가 파킨슨병의 병리 생리학적 특징이라는 사실이 입증되었습니다.
니코틴아미드는
다양한 대사 경로를 통해
니코틴아미드 아데닌 디뉴클레오티드(NAD, 산화 형태: NAD+, 환원 형태: NADH)의
생합성에 참여합니다[27].
NADH는
티로신을 수산화하여
도파민을 생성하는 티로신 하이드 록실화 효소에서 작용하는
테트라하이드로비옵테린을 보조하는 필수 보조 인자이며,
NADH 결핍은 PD에서 흔합니다 [28].
둘째, NADH는
ATP 합성에서 미토콘드리아 복합체 I의 생리적 기능에 필수 불가결하며,
해당 기능 장애는 PD 환자 및 동물 모델에 관여합니다 [15, 29, 30].
https://kr.iherb.com/pr/life-extension-nad-cell-regenerator-100-mg-30-vegetarian-capsules/62199
니코틴아미드 모노뉴클레오티드(NMN)는
NAD+의 주요 전구체 중 하나입니다.
https://kr.iherb.com/pr/california-gold-nutrition-nmn-powder-300-mg-1-06-oz-30-g/112136
이전의 시험관 연구에서 학자들은 로테논으로 처리된 PC12 세포를 사용하여 PD의 세포 모델을 확립했으며, 로테논으로 처리된(0.5 μm) PC12 세포에서 NMN(0.1mM 또는 1mM) 치료가 유의하게 높은 생존율과 관련이 있다는 것을 발견했습니다. NMN은 PD의 세포 모델에서 NAD+ 및 ATP의 세포 내 수준을 향상시키는 것으로 추정됩니다 [31].
또한
니코틴아마이드는
산화 스트레스를 억제하여
신경 보호 역할을 할 수 있습니다.
1-메틸-4-페닐-1,2,3,6-테트라하이드로피리딘-(MPTP-) 유도 PD 마우스 모델에서
니코틴아마이드(500 mg/kg)를 아급성(30 mg/kg/d 5일간) MPTP 투여 전에 주사했습니다.
이 연구에 따르면 MPTP와 니코틴아마이드의 병용 치료는 MPTP 단독 치료와 비교하여 운동 활성을 유의하게 개선했습니다. 니코틴아마이드 투여는 MPTP로 인한 도파민 고갈을 유의하게 약화시켰습니다(47.11 ± 21.06 vs. 12.77 ± 8.06). 한편, 니코틴아마이드 전처리는 MPTP에 의한 젖산 탈수소효소(LDH)와 NOS 활성을 현저히 억제하여 산화 스트레스를 예방하고 산화 손상을 완화했습니다.
시르투인(SIRT)은
중요한 생물학적 과정에 관여하는
NAD+ 의존성 단백질 탈아세틸화 효소입니다[32].
최근 시르투인 5(SIRT5)가 상당한 주목을 받고 있습니다. Liu 등은 MPTP로 유도된 마우스 PD 모델에서 SIRT5의 역할을 조사했습니다[33]. 그들은 SIRT5의 결실이 PD 모델에서 운동 결손, 흑질(SNc)의 좁은 부분의 흑질 도파민성 변성, 미토콘드리아 항산화 활동을 악화시킨다는 사실을 발견했습니다. 이러한 연구 결과는 PD의 치료 전략에 대한 새로운 통찰력을 제공합니다. 그러나 니코틴아미드의 보호 효과는 여전히 논란의 여지가 있으며, PD에서 비타민 B3의 생물학적 기능을 명확히 밝히기 위해서는 추가 연구가 필요합니다.
3.3. Clinical Studies regarding Vitamin B3 in PD
Current existing clinical studies have shown that a high-niacin diet can reduce the risk of PD [34, 35]. A previous case report also demonstrated that oral niacin (500 mg twice daily for three months) significantly improved rigidity and bradykinesia in a patient with idiopathic PD, though the original purpose was to treat hypertriglyceridemia; after the cessation of oral niacin due to obvious adverse effects (unacceptable nightmares and skin rash), the symptoms of rigidity and bradykinesia relapsed [36]. However, other studies failed to notice the remarkable clinical efficacy [37, 38]. Therefore, more clinical observations are warranted to verify the efficacy as well as side effects of niacin in PD.
3.3. PD에서 비타민 B3에 관한 임상 연구
기존의 기존 임상 연구에 따르면
고니아신 식단은
파킨슨병의 위험을 줄일 수 있는 것으로 나타났습니다 [34, 35].
이전의 사례 보고에서도
경구용 니아신(500mg을 3개월간 1일 2회 복용)이
특발성 PD 환자의 강직과 서동증을 유의하게 개선한 것으로 나타났는데,
원래 목적은 고중성지방혈증 치료였지만
명백한 부작용(견딜 수 없는 악몽과 피부 발진)으로 인해
경구용 니아신을 중단한 후
강직과 서동증 증상이 재발했습니다 [36].
그러나 다른 연구에서는 놀라운 임상 효능을 발견하지 못했습니다 [37, 38]. 따라서 PD에서 니아신의 효능과 부작용을 확인하기 위해서는 더 많은 임상 관찰이 필요합니다.
4. Vitamin C and PD
Vitamin C (ascorbic acid) is another water-soluble, essential vitamin, which is widely distributed in various tissues. This nutriment is abundant in vegetables, fresh fruits, and animal livers. Vitamin C contains two molecular subforms in organisms: the reduced form (ascorbic acid (AA)) and the oxidized form (dehydroascorbic acid (DHA)). Deficiency of vitamin C is common, especially in children and elderly. A long-term lack of vitamin C can cause scurvy. Vitamin C is very important for the physiological function of the nervous system and the antioxidant function by inhibiting oxidative stress, reducing lipid peroxidation, and scavenging free radicals [39]. Moreover, it is also involved in many nonoxidative stress processes, such as synthesis of collagen, cholesterol, carnitine, catecholamines, amino acids, and some peptide hormones [40, 41].
Dopamine metabolism can produce oxidative stress products, which in return induce accumulation of abnormal proteins in PD [42]. Vitamin C has potentials for the treatment of PD considering the following reasons. Firstly, vitamin C is mainly distributed in areas that are rich in neurons [43, 44]. Secondly, vitamin C can be transported to the brain by SVCT2 (vitamin C transporter type 2) [45], and DHA can be transported to the brain by GLUT1 (glucose transporter type 1) and GLUT3 (glucose transporter type 3) [46].
4. 비타민 C와 PD
비타민 C(아스코르브산)는
다양한 조직에 널리 분포하는
또 다른 수용성 필수 비타민입니다.
이 영양소는 채소, 신선한 과일, 동물의 간에 풍부하게 함유되어 있습니다.
비타민 C는
환원된 형태(아스코르브산(AA))와
산화된 형태(디하이드로아스코르브산(DHA))의
두 가지 분자 하위 형태를 유기체에 포함하고 있습니다.
비타민 C 결핍은 특히
어린이와 노인에게 흔합니다.
비타민 C가 장기간 부족하면
괴혈병이 발생할 수 있습니다.
비타민 C는
산화 스트레스를 억제하고
지질 과산화를 줄이며
활성 산소를 제거하여
신경계의 생리 기능과 항산화 기능에 매우 중요합니다 [39].
또한
콜라겐,
콜레스테롤,
카르니틴,
카테콜아민,
아미노산 및 일부 펩타이드 호르몬의 합성과 같은
많은 비산화 스트레스 과정에도 관여합니다 [40, 41].
도파민 대사는
산화 스트레스 생성물을 생성하여
PD에 비정상적인 단백질의 축적을 유도할 수 있습니다 [42].
비타민 C는 다음과 같은 이유를 고려할 때
PD 치료에 잠재력이 있습니다.
첫째, 비타민 C는
주로 뉴런이 풍부한 영역에 분포합니다 [43, 44].
둘째, 비타민 C는
SVCT2(비타민 C 수송체 2형)[45]에 의해
뇌로 운반될 수 있으며,
DHA는 GLUT1(포도당 수송체 1형)과
GLUT3(포도당 수송체 3형)에 의해 뇌로 운반될 수 있습니다[46].
4.1. Possible Neuroprotective Mechanisms of Vitamin C in PD
There is evidence that ascorbic acid can protect against both levodopa toxicity and the MPTP neurotoxicity [47, 48]. Vitamin C can increase the production of dihydroxyphenylalanine (DOPA). Seitz et al. noted overproduction of DOPA in a dose-dependent manner after incubation of the human neuroblastoma cell line SK-N-SH with ascorbic acid (100-500 mM) for 2 hours. Additionally, the gene expression of tyrosine hydroxylase increased threefold after incubation with ascorbic acid (200 mM) for 5days. The scholars speculated that ascorbic acid may be effective in the treatment of early-stage PD [49].
Vitamin C can improve the absorption of levodopa in elderly PD patients with a poor levodopa bioavailability [50]. Previous studies showed that ascorbic acid can reduce the levodopa dosage under the premise of equal efficacy [51]. Combination of anti-PD drugs and vitamin C may be more effective for alleviating the symptoms of PD.
Vitamin C is essential for the brain development. A study showed that ascorbic acid treatment can promote a 10-fold increase of dopaminergic differentiation in CNS precursor cells derived from the E12 rat mesencephalon [52]. Soon after, another in vitro study also reported that AA can stimulate the CNS precursor cells differentiating into CNS neurons and glia [53]. Recently, He et al. proposed that vitamin C can greatly enhance the embryonic midbrain neural stem cells differentiating into midbrain dopaminergic neurons in vitro. Vitamin C induces the gain of 5-hydroxymethylcytosine (5HMC) and loss of H3K27m3 in dopaminergic phenotype gene promoters, which are catalysed by ten-eleven translocation 1 methylcytosine dioxygenase 1 (TET1) and histone H3K27 demethylase (JMJD3), respectively. However, subsequent TET1 and JMJD3 knockdown/inhibition experiments did not show this effect of vitamin C, and the epigenetic role of vitamin C may be associated with the midbrain dopaminergic neuron development [54, 55].
4.1. PD에서 비타민 C의 가능한 신경 보호 메커니즘
아스코르브산이
레보도파 독성과 MPTP 신경 독성을 모두 예방할 수 있다는 증거가 있습니다 [47, 48].
비타민 C는
디하이드록시페닐알라닌(DOPA)의 생성을 증가시킬 수 있습니다.
Seitz 등은 인간 신경모세포종 세포주 SK-N-SH를 아스코르브산(100-500mM)으로 2시간 동안 배양한 후 용량 의존적인 방식으로 DOPA의 과잉 생산에 주목했습니다. 또한 티로신 하이드 록 실라 제의 유전자 발현은 아스코르브 산 (200mM)으로 5 일 동안 배양 한 후 3 배 증가했습니다. 학자들은 아스코르브산이 초기 단계의 PD 치료에 효과적일 수 있다고 추측했습니다 [49].
비타민 C는 레보도파 생체 이용률이 낮은
노인 PD 환자에서
레보도파의 흡수를 개선할 수 있습니다 [50].
이전 연구에 따르면
아스코르브 산은 동일한 효능을 전제로
레보도파 복용량을 줄일 수 있습니다 [51].
항 PD 약물과 비타민 C의 병용은
PD 증상 완화에 더 효과적일 수 있습니다.
비타민 C는
두뇌 발달에 필수적입니다.
한 연구에 따르면 아스코르브산 처리는 E12 쥐의 중뇌에서 유래한 CNS 전구세포에서 도파민 분화를 10배 증가시킬 수 있는 것으로 나타났습니다 [52]. 얼마 지나지 않아 또 다른 시험관 내 연구에서도 AA가 CNS 뉴런과 신경교세포로 분화되는 CNS 전구세포를 자극할 수 있다고 보고했습니다 [53]. 최근 He 등은 비타민 C가 시험관 내에서 중뇌 도파민성 뉴런으로 분화하는 배아 중뇌 신경 줄기 세포를 크게 향상시킬 수 있다고 제안했습니다. 비타민 C는 도파민성 표현형 유전자 프로모터에서 5- 하이드 록시 메틸 시토신 (5HMC)의 증가와 H3K27m3의 손실을 유도하며, 이는 각각 10-11 전위 1 메틸 시토신 디 옥시 효소 1 (TET1)과 히스톤 H3K27 탈 메틸 효소 (JMJD3)에 의해 촉매된다. 그러나 후속 TET1 및 JMJD3 녹다운/억제 실험에서는 비타민 C의 이러한 효과가 나타나지 않았으며, 비타민 C의 후성유전학적 역할은 중뇌 도파민 신경세포 발달과 관련이 있을 수 있습니다[54, 55].
4.2. Clinical Studies regarding Vitamin C in PD
Although vitamin C has many potential positive effects on PD, the serum level of vitamin C in patients with PD remains controversial [56, 57]. Noteworthily, the vitamin C level in lymphocytes has been found significantly lower in patients with severe PD [58]. Theoretically, vitamin C supplementation may be beneficial for the treatment of PD. A cohort study involving 1036 patients with PD supported this hypothesis, which found that dietary vitamin C intake significantly reduced the risk of PD, but this effect is invalid for a 4-year-lag analysis [59]. Controversially, many studies did not support that vitamin C supplementation can reduce the risk of PD [10, 60, 61]. We speculate this contradiction may be related to the timing of vitamin application.
4.2. PD의 비타민 C 관련 임상 연구
비타민 C는
pd에 많은 잠재적인 긍정적인 효과를 가지고 있지만,
pd 환자의 혈청 비타민 C 수치는 여전히 논란의 여지가 있습니다 [56, 57].
주목할 만한 점은 림프구의 비타민 C 수치가 중증 PD 환자에서 현저히 낮은 것으로 밝혀졌습니다 [58]. 이론적으로 비타민 C 보충제는 pd치료에 도움이 될 수 있습니다. PD 환자 1036명을 대상으로 한 코호트 연구에 따르면 식이 비타민 C 섭취가 PD의 위험을 크게 감소시킨다는 가설이 뒷받침되었지만, 4년 시차를 둔 분석에서는 이 효과가 유효하지 않습니다 [59]. 논란의 여지가 있지만, 많은 연구에서 비타민 C 보충제가 PD의 위험을 줄일 수 있다는 것을 뒷받침하지 못했습니다 [10, 60, 61]. 이러한 모순은 비타민 복용 시기와 관련이 있을 수 있다고 추측합니다.
5. Vitamin E and PD
Vitamin E is a fat-soluble vitamin with high antioxidant properties. Natural vitamin E includes two subgroups: tocopherols and tocotrienols; and they can further be divided into four lipophilic molecules, respectively: α-, β-, γ-, and δ-tocopherol (αT, βT, γT, and δT) and α-, β-, γ-, and δ-tocotrienol (αTE, βTE, γTE, and δTE). The major difference between tocopherols and tocotrienols is the side chain. Tocopherols have a saturated phytyl tail, while tocotrienols possess an unsaturated isoprenoid side chain [62]. Because of this unsaturated side chain, the tocotrienol is superior to the tocopherol as an antioxidant by increasing the molecular mobility through lipid membranes and by accepting electrons readily. Overt vitamin E deficiency is relatively rare, mainly in infants and premature babies.
In addition to its potent antioxidant capacity, vitamin E is involved in many physiological processes such as immune function [63], cognitive function, physical performance [64, 65], and regulation of gene expression. In humans, deficiency of vitamin E is clinically characterized by peripheral neuropathy, ataxia, and anemia [66, 67].
5. 비타민 E와 PD
비타민 E는
항산화 효과가 높은 지용성 비타민입니다.
천연 비타민 E에는
토코페롤과 토코트리에놀의 두 가지 하위 그룹이 있으며,
이들은 각각 α-, β-, γ- 및 δ-토코페롤(αT, βT, γT 및 δT)과
α-, β-, γ- 및 δ-토코트리에놀(αTE, βTE, γTE 및 δTE)의
네 가지 친유성 분자로 다시 나눌 수 있습니다.
토코페롤과 토코트리에놀의 가장 큰 차이점은 측쇄입니다.
토코페롤은 포화 피틸 꼬리를 가지고 있는 반면 토코트리에놀은 불포화 이소프레노이드 측쇄를 가지고 있습니다[62]. 이 불포화 측쇄로 인해 토코트리에놀은 지질막을 통해 분자 이동성을 높이고 전자를 쉽게 받아들임으로써 항산화제로서 토코페롤보다 우수합니다. 명백한 비타민 E 결핍은 주로 영유아와 미숙아에서 비교적 드물게 발생합니다.
비타민 E는 강력한 항산화 능력 외에도
면역 기능[63],
인지 기능,
유전자 발현 조절과 같은 많은 생리학적 과정에 관여합니다.
인간의 경우 비타민 E 결핍은 임상적으로
말초 신경병증, 운동 실조증, 빈혈을 특징으로 합니다[66, 67].
5.1. Possible Neuroprotective Mechanisms of Vitamin E in PD
Unilateral 6-hydroxydopamine (6-OHDA) injections into the striatum can cause circling behaviours and biochemical abnormalities in rats. Cadet et al. found that pretreatment with either D-alpha-tocopherol or all-racemic-alpha-tocopherol significantly attenuated these pathological changes [68]. Roghani and Behzadi [69] and Sharma and Nehru [70] also demonstrated the similar phenomenon in 6-OHDA-induced PD models and in rotenone-induced PD models, respectively. However, some studies have shown that vitamin E did not completely protect dopaminergic neurons from MPTP-mediated damage in PD models [71, 72]. The protective effects of vitamin E may be achieved through preventing oxidative stress in cells and inhibiting apoptosis. Moreover, one study has found that tocotrienol participates not only in antioxidant stress but also in estrogen receptor beta (ERβ) signal transduction [73]. Then, Nakaso's team demonstrated a protective effect of vitamin E via this signaling pathway. Firstly, they reported that γ-tocotrienol/δ-tocotrienol exerts neuroprotective effects through the ERβ-PI3K/Akt signaling pathways in SH-SY5Y cells by resisting 1-methyl-4-phenylpyridiniumion- (MPP+-) induced toxicity [74]. Secondly, they verified this mechanism in a mouse model of PD. Meanwhile, they found δ-tocotrienol administration can reduce the loss of dopaminergic neurons in the substantia nigra and ER inhibitors can attenuate this neuroprotective effect [75]. These findings indicate vitamin E may be potential therapeutic agents for PD.
5.1. PD에서 비타민 E의 가능한 신경 보호 메커니즘
선조체에
일방적으로 6-하이드록시도파민(6-OHDA)을 주입하면
쥐의 원형 행동과 생화학적 이상이 발생할 수 있습니다.
Cadet 등은 D-알파-토코페롤 또는 전-알파-토코페롤로 전처리하면 이러한 병리학적인 변화가 현저히 약화된다는 사실을 발견했습니다[68]. Roghani와 Behzadi [69]와 Sharma와 Nehru [70]도 각각 6-OHDA 유도 PD 모델과 로테논 유도 PD 모델에서 유사한 현상을 입증했습니다. 그러나 일부 연구에서는 비타민 E가 PD 모델에서 도파민성 뉴런을 MPTP 매개 손상으로부터 완전히 보호하지 못한다는 사실이 밝혀졌습니다 [71, 72].
비타민 E의 보호 효과는
세포의 산화 스트레스를 예방하고
세포 사멸을 억제함으로써 달성될 수 있습니다.
또한 한 연구에 따르면 토코트리에놀은 항산화 스트레스뿐만 아니라 에스트로겐 수용체 베타(ERβ) 신호 전달에도 관여하는 것으로 밝혀졌습니다 [73]. 그런 다음 나카소 박사팀은 이 신호 전달 경로를 통해 비타민 E의 보호 효과를 입증했습니다.
첫째, γ-토코트리에놀/δ-토코트리에놀은 1-메틸-4-페닐피리디늄이온(MPP+-)에 의한 독성에 저항함으로써 SH-SY5Y 세포에서 ERβ-PI3K/Akt 신호 경로를 통해 신경 보호 효과를 발휘한다는 사실을 보고했습니다[74].
두 번째로, 연구진은 이 메커니즘을 파킨슨병 마우스 모델에서 확인했습니다. 한편 δ-토코트리에놀을 투여하면 흑질에서 도파민 신경세포의 손실을 줄일 수 있으며 ER 억제제는 이러한 신경 보호 효과를 약화시킬 수 있다는 것을 발견했습니다 [75]. 이러한 연구 결과는 비타민 E가 파킨슨병의 잠재적 치료제가 될 수 있음을 시사합니다.
5.2. Clinical Studies regarding Vitamin E in PD
The DATATOP (Deprenyl and Tocopherol Antioxidative Therapy of Parkinsonism) experiment is a multicentre-controlled clinical trial to investigate the long-term efficacy of treatment with deprenyl and/or copherol (vitamin E) and to explore whether it is possible to extend the time before the application of levodopa treatment. At 28 US and Canadian sites, 800 eligible patients with untreated early-stage PD were enrolled in DATATOP and randomized to four groups: (1) deprenyl 10 mg/d, (2) copherol 2000 IU/d, (3) placebo-controlled, and (4) deprenyl 10 mg/d and copherol 2000 IU/d. Deprenyl can delay the development of functional disorders, delay the application of levodopa, and improve motor symptoms, but vitamin E is disappointing [76]. Similarly, another two population-based studies also did not find the association between vitamin E intake and risk of PD [10, 77].
However, a large community-based study showed that high intake of dietary vitamin E (10 mg/day) may reduce the occurrence of PD [78]. Another pilot trail suggests that long-term treatment with vitamin E may delay the use of levodopa in patients with PD [79]. Further research is needed to verify these results.
Although there seems to be no difference in the level of alpha-tocopherol (vitamin E) in serum, cerebrospinal fluid, and brains between PD and normal controls [80–82], there is evidence showing that high-dose vitamin E (2000 IU/day) can significantly elevate the vitamin E level in cerebrospinal fluid [83]. At present, the protective mechanism of vitamin E in PD is still unclear and may be related to the strong antioxidant effect of vitamin E. Further research is needed to determine whether vitamin E can be used as a potential treatment for PD.
5.2. 파킨슨병에 대한 비타민 E 관련 임상 연구
데프레닐 및/또는 토코페롤(비타민 E) 치료의 장기적인 효능을 조사하고 레보도파 치료 적용 전 시간을 연장할 수 있는지를 알아보기 위한 다기관 대조 임상시험인 DATATOP(파킨슨병의 데프레닐 및 토코페롤 항산화 치료) 실험이 진행 중입니다. 미국과 캐나다의 28개 기관에서 치료 경험이 없는 초기 파킨슨병 환자 800명을 대상으로 (1) 데프레닐 10 mg/d, (2) 코페롤 2000 IU/d, (3) 위약 대조군, (4) 데프레닐 10 mg/d 및 코페롤 2000 IU/d의 네 그룹으로 무작위 배정하여 DATATOP에 등록했습니다. 데프레닐은 기능 장애의 발생을 지연시키고 레보도파의 적용을 지연시키며 운동 증상을 개선할 수 있지만, 비타민 E는 실망스러운 결과를 보였습니다 [76]. 마찬가지로, 또 다른 두 건의 인구 기반 연구에서도 비타민 E 섭취와 파킨슨병 위험 사이의 연관성을 찾지 못했습니다 [10, 77].
그러나 대규모 지역사회 기반 연구에 따르면 식이 비타민 E(하루 10 mg/일)를 많이 섭취하면 PD 발생을 줄일 수 있는 것으로 나타났습니다 [78]. 또 다른 파일럿 연구에 따르면 비타민 E를 장기간 복용하면 PD 환자에서 레보도파 사용이 지연될 수 있다고 합니다[79]. 이러한 결과를 검증하려면 추가 연구가 필요합니다.
혈청, 뇌척수액 및 뇌의 알파-토코페롤(비타민 E) 수준은 PD와 정상 대조군 간에 차이가 없는 것으로 보이지만[80-82], 고용량 비타민 E(2000 IU/일)가 뇌척수액의 비타민 E 수준을 크게 높일 수 있다는 증거가 있습니다[83]. 현재 PD에서 비타민 E의 보호 메커니즘은 아직 명확하지 않으며 비타민 E의 강력한 항산화 효과와 관련이 있을 수 있습니다. 비타민 E가 PD의 잠재적 치료제로 사용될 수 있는지 여부를 결정하기 위해서는 추가 연구가 필요합니다.
6. Vitamin D and PD
Vitamin D, a steroid hormone, is crucial for calcium homeostasis and skeletal health. This nutriment mainly includes two forms: vitamin D2 and vitamin D3; the latter is endogenously produced when skin is exposed to UV-B rays from the sun. Both of the above forms are inactive, and they are transformed into the active form 1,25-dihydroxy vitamin D3 (1,25-(OH)2-D3) after being hydroxylated twice [84, 85]. 1,25-(OH)2-D3 were secreted into the blood system by the kidney, having a direct effect on gene regulation by binding to the nuclear vitamin D receptor (VDR) [86, 87]. Vitamin D deficiency is prevalent at all ages, especially in elderly. Vitamin D not only regulates the calcium homeostasis and skeletal health but also regulates the physiological and pathological processes, such as cell proliferation and differentiation, immunomodulatory, and antioxidative stress [88–90]. Children with a lack of vitamin D may suffer from rickets, and adults may develop osteomalacia. Additionally, vitamin D deficiency is also associated with cardiovascular diseases, muscle weakness, diabetes mellitus, cancers, and multiple sclerosis [91]. The relationship between vitamin D and PD has gradually attracted attention [92].
6.1. Possible Neuroprotective Mechanisms of Vitamin D in PD
VDR belongs to the intranuclear receptor superfamily, composing of eight coding exons and three alternative 5′ noncoding exons, spanning over 105 kb, on chromosome 12 [93]. The most widely studied biallelic polymorphic sites are BsmI, TaqI, ApaI, and FokI. Substantial researches have been carried out to explore the relationship between these allelic variations and PD. Kim et al. detected VDR gene BsmI polymorphisms in over 300 Korean individuals (85 PD and 231 controls). The frequency of VDR genotype bb was significantly increased in the PD patients (84.7%) than that in the controls (72.7%). The bb genotype was more common in PD patients with postural instability and gait difficulty than in the PD patients with tremor (94.3% vs. 75.6%) [94]. A meta-analysis showed that VDR BsmI and FokI polymorphisms were associated with the risk of PD [95], and VDR FokI genotype was associated with the severity and cognitive decline of PD [96, 97]. Muscular and motor impairments, which can seriously affect the motor behaviour, were found in the VDR-knockout mice [98], indicating that vitamin D may be involved in the pathogenesis of PD.
Glial cell line-derived neurotrophic factor (GDNF) is a protein that is essential for the maintenance and survival of dopaminergic neurons and can inhibit microglial activation [99]. Many animal studies showed that 1,25-(OH)2-D3 could enhance the endogenous GDNF expression in vitro and in vivo and inhibit the glial cell activation to protect dopaminergic neurons from immune inflammation [100–102].
Vitamin D3 can protect dopaminergic neurons against 6-hydroxydopamine-mediated neurotoxicity and improve the motor performance in the 6-hydroxydopamine-induced PD rat [103]. It may be related to vitamin D's properties of inhibiting oxidative stress and decreasing the production of reactive oxygen species and free radicals [104]. In addition, endothelial dysfunction may be associated with low vitamin D levels in patients with PD [105]. The definitive correlations between vitamin D and PD require more researches.
6.2. Clinical Studies regarding Vitamin D in PD
Substantial epidemiological and clinical studies suggest that vitamin D has a positive effect on PD. In a cohort study, over 7000 Finnish's serum samples were collected for measuring the 25-hydroxy vitamin D level, and meanwhile, the occurrence of PD was instigated over a 30-year follow-up period. The results showed that individuals with higher serum vitamin D concentrations had a lower risk of PD [106]. Evatt et al. also noted consistent findings [107].
As mentioned above, vitamin D3 can be endogenously synthesized upon sunlight exposure in the skin. In a large case-control study of Danish men, involving 3819 PD patients and 19,282 controls, the scholars proposed that men working outdoors have a lower risk of PD [108]. Another nationwide ecologic study in France also suggests that vitamin D levels are negatively correlated with the risk of PD, but this result needs taking ages into account [109]. Wang et al. not only demonstrated a positive correlation between serum 25-hydroxy vitamin D and sunlight exposure but also noted that lower serum levels of 25-hydroxy vitamin D and sunlight exposure can increase the risk of PD [110].
Furthermore, PD patients with lower 25-hydroxy vitamin D levels may exhibit more severe symptoms compared with normal controls [111, 112]. Unsurprisingly, a randomized, double-blind, placebo-controlled trial found that vitamin D3 supplementation (1200 IU/day for 12 months) significantly prevented the deterioration of PD [113].
7. Conclusion
In summary, vitamins may play a protective role in PD. Among the fat-soluble vitamins, we briefly summarized the effects of vitamin E and vitamin D. At present, although many studies have shown that vitamin E supplementation can reduce the risk of Parkinson's disease (Table 1), the DATATOP study has showed that vitamin E supplementation is ineffective in Parkinson's disease (Table 2). Many noninterventional studies found that the high levels of serum vitamin D can reduce the risk of PD (Table 1), and several clinical intervention trials also proposed that vitamin D supplementation can attenuate the deterioration of the Parkinson's disease and reduce the occurrence of fractures in patients with PD (Table 2). Among the water-soluble vitamins, we elaborated the functions of vitamin B3 and vitamin C. There is still a paucity of clinical evidence for determining the pros and cons of vitamin B3 in PD (Table 2). Vitamin C is vital to the human organism, and it can improve levodopa absorption in elderly PD patients (Table 2); current epidemiological evidence is still insufficient to establish a correlation between the serum level or dietary intakes of vitamin C and the risk of PD (Table 1). Although there have been many researches on the relationship between vitamins and PD (Tables (Tables111–3), there is still lack of a clinical intervention trial explicitly confirming that vitamin supplementation can reduce the incidence of PD and prevent the progression of the disease. Moreover, the individual physical and chemical properties, absorption rate, and bioavailability of vitamins may affect the efficacy. Further studies are still needed to clarify the potentials of vitamins for the treatment of PD.
Table 1
The other clinical study of vitamins and Parkinson's disease.
VitaminsAuthorsType of studyPatients/controlsConclusions
Vitamin B3 | Abbott et al. [37] | A Honolulu-Asia Aging Study in Japanese-American | Total 8006 and observed 137 PD | Niacin has no obvious relationship with clinical PD |
Johnson et al. [38] | A case-control study in US | 126/432 | Niacin has no relationship with PD | |
Fall et al. [34] | A case-control study in Sweden | 113/263 | High-niacin diet can reduce the risk of PD | |
Hellenbrand et al. [35] | A case-control study in German | 342/342 | PD patients with lower intake of niacin than controls | |
Vitamin C | Yang et al. [61] | A prospective study in Sweden | Total 84,774 and observed 1329 PD cases | Intake of vitamin C has a negative correlation with PD risk in women at borderline significance (P = 0.04) |
Hughes et al. [59] | A prospective study in American | Total 129,422 and observed 1036 PD cases | Intake of vitamin C has no relationship with PD risk | |
Ide et al. [58] | A case-case study in Japan | 62 PD | The severe PD patients with significantly lower lymphocyte vitamin C levels (P < 0.01) | |
Miyake et al. [60] | A case-control study in Japan | 249/368 | Intake of vitamin C has no relationship with PD risk | |
Zhang et al. [10] | A prospective study in US | Total 124,221 and observed 371 PD cases | Intake of vitamin C has no relationship with PD risk | |
Férnandez-Calle et al. [56] | A case-control study in Spain | 63/63 | Vitamin C has no relationship with PD | |
King et al. [127] | A case-control study in United States | 27/16 | Vitamin C was higher in PD groups | |
Vitamin E | Yang et al. [61] | A prospective study in Sweden | Total 84,774 and observed 1329 PD cases | Dietary intake of vitamin E has negative correlation with the incidence of PD in women (P = 0.02) |
Hughes et al. [59] | A prospective study in American | Total 129,422 and observed 1036 PD cases | Vitamin E has no relationship with PD risk | |
Miyake et al. [60] | A case-control study in Japan | 249/368 | Vitamin E significantly reduced the risk of PD | |
Zhang et al. [10] | A prospective study in US | Total 124,221 and observed 371 PD cases | Intaking foods containing more vitamin E can reduce the risk of Parkinson's disease | |
Molina et al. [82] | A case-control study in Spain | 34/47 | CSF and serum vitamin E levels have no difference between two groups | |
de Rijk et al. [78] | A cross-sectional study in Netherlands | 5342 individuals including 31 PD cases | Intaking 10 mg dietary vitamin E daily may reduce the risk of PD | |
Logroscino et al. [77] | A case-control study in USA | 110/287 | Vitamins A, C, and E were not associated with PD | |
Férnandez-Calle et al. [80] | A case-control study in Spain | 42/42 | Serum levels of alpha-tocopherol (vitamin E) have no difference between two groups | |
Vitamin D | Kim et al. [128] | A prospective, observational study in Korea | 39 PD cases | The level of vitamin D might impact the olfactory dysfunction in PD |
Sleeman et al. [112] | A prospective observational study in England | 145/94 | Serum 25(OH)D concentrations are often lower in PD patients than controls and relate to the severity of motor symptoms | |
Wang et al. [110] | A case-control study in China | 201/199 | The serum 25(OH)D and sunlight exposure inversely correlated with PD occurrence | |
Shrestha et al. [129] | A prospective study in USA | Total 12,762 participants and observed 67 PD cases | This study did not suggest that the vitamin D can reduce the risk of PD | |
Liu and Zhang [111] | A case-control study in China | 229/120 | The 25(OH) D levels may be inversely associated with the PD severity | |
Lin et al. [130] | A case-control study in China | 700/792 | They have not found the associations between the genetic variants of VDR and PD occurrence | |
Zhu et al. [131] | A case-control study in China | 209/210 | Outdoor activity and total vitamin D intake may reduce the risk of PD | |
Petersen et al. [132] | A case-control study in Denmark | 121/235 | They have not found the association between PD and vitamin D polymorphisms and/or 25(OH)D levels | |
Török et al. [133] | A case-control study in Hungary | 100/109 | It showed the association between the FokI C allele and PD | |
Lv et al. [134] | A case-control study in China | 483/498 | The study did not support the relationship between VDR gene and PD | |
Peterson et al. [135] | A cross-sectional, observational study in USA | 40 PD | Serum vitamin D levels are inversely related to the severity of Parkinson's disease and play an important role in balance of PD | |
Suzuki et al. [96] | A prospective cohort study in Japan | 137 PD | The 25-hydroxyvitamin D levels and the vitamin D receptor FokI CC genotype may be associated with the severity of the PD | |
Kenborg et al. [108] | A case-control study in Denmark | 3819/19,282 | This study supports that working outdoors can reduce the risk of PD | |
Evatt et al. [136] | A survey study in USA | 199 PD (from DATATOP) | Vitamin D insufficiency is very common in early PD patients | |
Miyake et al. [137] | A case-control study in Japan | 249/368 | The study showed that vitamin D was not related to the PD | |
Knekt et al. [106] | The Mini-Finland Health Survey in Finland | Total 3173 and observed 50 PD cases | The serum vitamin D concentrations were inversely correlated with the risk of PD | |
Kim et al. [94] | A case-control study in Korea | 85/231 | Vitamin D receptor gene polymorphism was associated with the PD |
Table 2
The clinical intervention trial of vitamins and Parkinson's disease.
VitaminsAuthorsPatientsTreatmentConclusions
Vitamin C | Nagayama et al. [50] | 67 elderly PD patients | 200 mg ascorbic acid | Ascorbic acid can improve levodopa absorption in elderly PD patients |
Vitamin E | Parkinson Study Group (DATATOP study) [76] | 800 untreated and early PD patients | Deprenyl 10 mg/d and/or tocopherol (vitamin E) 2000 IU/d | There was no effect of tocopherol on PD |
Parkinson Study Group (DATATOP study) [124] | 800 untreated and early PD patients | Deprenyl 10 mg/d and/or tocopherol (vitamin E) 2000 IU/d | Alpha-tocopherol did not improve clinical features in patients with Parkinson's disease | |
Vatassery et al. (DATATOP study) [83] | n = 18 (vitamin E group)/n = 5 (placebo group) | Tocopherol (vitamin E) 2000 IU/d | Treatment with vitamin E significantly increased the alpha-tocopherol concentrations in cerebrospinal fluid | |
Taghizadeh et al. [125] | n = 30 (vitamin E group)/n = 30 (placebo group) | 1000 mg omega-3 fatty acids plus 400 IU vitamin or placebo | Omega-3 and vitamin E cosupplementation in PD patients improved UPDRS compared with the placebo | |
Vitamin D | Suzuki et al. [113] | n = 56 (vitamin D3 group)/n = 58 placebo group) | Vitamin D3 1200 IU/d or placebo for 12 months | Vitamin D3 prevented the deterioration of the PD and especially patients with FokI TT genes |
Sato et al. [126] | n = 43 (vitamin D group)/n = 43 (placebo group) | 1α(OH)D3 1 μg/d or placebo for 12 months | 1alpha-hydroxyvitamin D3 supplements can reduce the risk of hip and other nonvertebral fractures in PD patients |
Table 3
The basic study of vitamins in PD.
VitaminAuthorsObject of studyTreatmentConclusions
Vitamin B3 (nicotinamide) | Lu et al. [31] | Rotenone-PC12 cells | NMN (0.1 mM, 1.0 mM, 5 mM, and 10 mM) coculture | Attenuated apoptosis and improved energy metabolism |
Xu et al. [114] | MPTP-C57BL/6 mice | 500 mg/kg/day for 5 days i.p. | Nicotinamide can alleviate MPTP-induced damage to dopaminergic neurons through antioxidant stress | |
Jia et al. [115] | MPP(+)-SK-N-MC human neuroblastoma cells and alpha-synuclein transgenic Drosophila PD model | Nicotinamide concentration (21, 51, 101, 301, and 501 mg/L and 3, 15, 30, and 60 mg/100 g) | High doses of nicotinamide can reduce oxidative stress and improve mitochondrial function | |
Anderson et al. [116] | MPTP-adult male C57Bl/6 mice | Nicotinamide (125, 250, or 500 mg/kg i.p.) | Recovered the striatal DA levels and SNc neurons after accepting nicotinamide | |
Vitamin C (ascorbic acid) | Khan et al. [117] | PD Drosophila model | L-Ascorbic acid (AA, 11.35 × 10−5 M, 22.71 × 10−5 M, 45.42 × 10−5 M, and 68.13 × 10−5 M for 21 days) | Except 11.35 × 10−5 M, other concentrations of AA attenuated the loss of climbing ability of PD model flies in a dose-dependent manner |
Yan et al. [52] | Mesencephalic precursors from the E12 rat | Ascorbic acid (0.1 μM, 1 μM, 10 μM, 100 μM, and 1 mM) | Ascorbic acid promoted the dopaminergic differentiation | |
Seitz et al. [49] | Human neuroblastoma cell line SK-N-SH | Short-term incubation (100–500 μM for 2 h) and long-term incubation (200 μM for 5 days) | Ascorbic acid increased the DOPA production and tyrosine hydroxylase gene expression | |
Pardo et al. [47] | Human neuroblastoma cell NB69 | 10−3 M ascorbic acid or 23 and 115 × 10−3 M alpha-tocopherol | Ascorbic acid prevents the levodopa toxicity and quinone formation, but alpha-tocopherol did not | |
Sershen et al. [48] | MPTP-BALB/cBy mice | Ascorbic acid 100 mg/kg i.p. | Ascorbic acid may protect against the MPTP neurotoxicity | |
Vitamin E | Nakaso et al. [75] | MPTP-C57BL/6 mice | δ-Tocotrienol (100 μg/kg for 4 days, p.o.) | δ-Tocotrienol administration inhibited the loss of dopaminergic neurons and improved the motor performance |
Sharma and Nehru [70] | Rotenone-Sprague-Dawley rats | Vitamin E (100 IU/kg/day for 35 days i.m.) | Vitamin E administration significantly improved locomotor activity and increased the dopamine level, GSH, and SOD | |
Ortiz et al. [118] | MPTP-C57BL/6 mice | Vitamin E (50 mg/kg/day p.o.) | Vitamin E administration has decreased the COX-2 activity, LPO, and nitrite/nitrate level | |
Pasbakhsh et al. [119] | 6-OHDA-rat | Alpha-tocopherol acid succinate (24 IU/kg, i.m.) | Vitamin E treatment can protect locus coeruleus neurons in the PD model | |
Roghani and Behzadi [69] | 6-OHDA – Sprague-Dawley rats | d-α-Tocopheryl acid succinate (24 I.U./kg, i.m.) | Vitamin E treatment improved the rotational behaviour and prevented the reduction of tyrosine hydroxylase-immunoreactive cells | |
Vitamin D | Lima et al. [120] | 6-OHDA-Wistar rats | 1,25-(OH)2D3 (1 μg/kg for 7 days or for 14 days, p.o.) | Vitamin D can protect the dopaminergic neurons by its anti-inflammatory and antioxidant properties |
Calvello et al. [121] | MPTP-male C57BL/6 N mice | Vitamin D (1 μg/kg for 10 days, i.g.) | Vitamin D administration attenuates neuroinflammation and dopaminergic neurodegeneration | |
Li et al. [122] | MPTP-C57BL/6 mice | Calcitriol (0.2, 1, and 5 μg/kg/day for 7 days p.o.) | Calcitriol can significantly attenuate the neurotoxicity induced by MPTP | |
Jang et al. [104] | Rotenone-SH-SY5Y cells | Calcitriol (0.0 μM, 0.63 μM, 1.25 μM, 2.5 μM, 5 μM, and 10 μM) | 1,25-Dyhydroxyvitamin D3 can induce the autophagy to protect against the rotenone-induced neurotoxicity | |
Cass et al. [123] | 6-OHDA-male Fischer-344 rats | Calcitriol (0.3 or 1.0 μg/kg/day for 8 days, i.h.) | Calcitriol can promote functional recovery of dopaminergic neurons and release of dopamine | |
Sanchez et al. [100] | 6-OHDA-Sprague-Dawley rats | 1,25(OH)(2)D(3) (1 μg/mL/kg/day for 7 days i.p.) | 1,25(OH)(2)D(3) treatment increased the GDNF protein expression and partially restored TH expression | |
Kim et al. [102] | 6-OHDA Sprague-Dawley rats and MPTP-C57BL/6 mice | 1,25-(OH)2D3 (1 μg/mL at 1 mL/kg/day for 7 days, i.p.) | 1,25-(OH)2D3 can inhibit the microglial activation and protect against nigrostriatal degeneration |
LPO: lipid peroxides; COX-2: ciclooxigenase-2; TH: tyrosine hydroxylase; i.p.: intraperitoneal; i.m.: intramuscular; i.g.: intragastrical; i.h.: hypodermic injection; p.o.: peros.
Acknowledgments
This work was supported by the crosswise project from the Ministry of Science and Technology of Jilin Province (Grant No. 3R2168713428) and the Natural Science Foundation of Jilin Province (Grant No. 20180101154JC).
Conflicts of Interest
The authors have no conflicts of interest to declare.
Authors' Contributions
Xiuzhen Zhao and Ming Zhang equally contributed to this study.
References
1. Gibb W. R., Lees A. J. The relevance of the Lewy body to the pathogenesis of idiopathic Parkinson’s disease. Journal of Neurology, Neurosurgery, and Psychiatry. 1988;51(6):745–752. doi: 10.1136/jnnp.51.6.745. [PMC free article] [PubMed] [CrossRef] [Google Scholar]
2. Khoo T. K., Yarnall A. J., Duncan G. W., et al. The spectrum of nonmotor symptoms in early Parkinson disease. Neurology. 2013;80(3):276–281. doi: 10.1212/WNL.0b013e31827deb74. [PMC free article] [PubMed] [CrossRef] [Google Scholar]
3. Postuma R. B., Aarsland D., Barone P., et al. Identifying prodromal Parkinson’s disease: pre-motor disorders in Parkinson’s disease. Movement Disorders. 2012;27(5):617–626. doi: 10.1002/mds.24996. [PubMed] [CrossRef] [Google Scholar]
4. Goswami P., Joshi N., Singh S. Neurodegenerative signaling factors and mechanisms in Parkinson’s pathology. Toxicology In Vitro. 2017;43:104–112. doi: 10.1016/j.tiv.2017.06.008. [PubMed] [CrossRef] [Google Scholar]
5. Spillantini M. G., Schmidt M. L., Lee V. M. Y., Trojanowski J. Q., Jakes R., Goedert M. α-Synuclein in Lewy bodies. Nature. 1997;388(6645):839–840. doi: 10.1038/42166. [PubMed] [CrossRef] [Google Scholar]
6. Hattingen E., Magerkurth J., Pilatus U., et al. Phosphorus and proton magnetic resonance spectroscopy demonstrates mitochondrial dysfunction in early and advanced Parkinson’s disease. Brain. 2009;132(12):3285–3297. doi: 10.1093/brain/awp293. [PubMed] [CrossRef] [Google Scholar]
7. Parker W. D., Jr, Parks J. K., Swerdlow R. H. Complex I deficiency in Parkinson’s disease frontal cortex. Brain Research. 2008;1189:215–218. doi: 10.1016/j.brainres.2007.10.061. [PMC free article] [PubMed] [CrossRef] [Google Scholar]
8. Sánchez-Hernández D., Anderson G. H., Poon A. N., et al. Maternal fat-soluble vitamins, brain development, and regulation of feeding behavior: an overview of research. Nutrition Research. 2016;36(10):1045–1054. doi: 10.1016/j.nutres.2016.09.009. [PubMed] [CrossRef] [Google Scholar]
9. Chawla J., Kvarnberg D. Hydrosoluble vitamins. Handbook of Clinical Neurology. 2014;120:891–914. doi: 10.1016/B978-0-7020-4087-0.00059-0. [PubMed] [CrossRef] [Google Scholar]
10. Zhang S. M., Hernan M. A., Chen H., Spiegelman D., Willett W. C., Ascherio A. Intakes of vitamins E and C, carotenoids, vitamin supplements, and PD risk. Neurology. 2002;59(8):1161–1169. doi: 10.1212/01.WNL.0000028688.75881.12. [PubMed] [CrossRef] [Google Scholar]
11. Sies H., Berndt C., Jones D. P. Oxidative stress. Annual Review of Biochemistry. 2017;86(1):715–748. doi: 10.1146/annurev-biochem-061516-045037. [PubMed] [CrossRef] [Google Scholar]
12. Kalia L. V., Lang A. E. Parkinson’s disease. The Lancet. 2015;386(9996):896–912. doi: 10.1016/S0140-6736(14)61393-3. [PubMed] [CrossRef] [Google Scholar]
13. Blesa J., Trigo-Damas I., Quiroga-Varela A., Jackson-Lewis V. R. Oxidative stress and Parkinson’s disease. Frontiers in Neuroanatomy. 2015;9:p. 91. doi: 10.3389/fnana.2015.00091. [PMC free article] [PubMed] [CrossRef] [Google Scholar]
14. Murphy M. P. How mitochondria produce reactive oxygen species. The Biochemical Journal. 2009;417(1):1–13. doi: 10.1042/BJ20081386. [PMC free article] [PubMed] [CrossRef] [Google Scholar]
15. Schapira A. H., Cooper J. M., Dexter D., Jenner P., Clark J. B., Marsden C. D. Mitochondrial complex I deficiency in Parkinson’s disease. The Lancet. 1989;333(8649):p. 1269. doi: 10.1016/s0140-6736(89)92366-0. [PubMed] [CrossRef] [Google Scholar]
16. Sian J., Dexter D. T., Lees A. J., et al. Alterations in glutathione levels in Parkinson’s disease and other neurodegenerative disorders affecting basal ganglia. Annals of Neurology. 1994;36(3):348–355. doi: 10.1002/ana.410360305. [PubMed] [CrossRef] [Google Scholar]
17. Gluck M. R., Zeevalk G. D. Inhibition of brain mitochondrial respiration by dopamine and its metabolites: implications for Parkinson’s disease and catecholamine-associated diseases. Journal of Neurochemistry. 2004;91(4):788–795. doi: 10.1111/j.1471-4159.2004.02747.x. [PubMed] [CrossRef] [Google Scholar]
18. Hare D. J., Double K. L. Iron and dopamine: a toxic couple. Brain. 2016;139(4):1026–1035. doi: 10.1093/brain/aww022. [PubMed] [CrossRef] [Google Scholar]
19. Sauberlich H. E. Implications of nutritional status on human biochemistry, physiology, and health. Clinical Biochemistry. 1984;17(2):132–142. doi: 10.1016/S0009-9120(84)90344-8. [PubMed] [CrossRef] [Google Scholar]
20. Mikkelsen K., Stojanovska L., Tangalakis K., Bosevski M., Apostolopoulos V. Cognitive decline: a vitamin B perspective. Maturitas. 2016;93:108–113. doi: 10.1016/j.maturitas.2016.08.001. [PubMed] [CrossRef] [Google Scholar]
21. Gehring W. Nicotinic acid/niacinamide and the skin. Journal of Cosmetic Dermatology. 2004;3(2):88–93. doi: 10.1111/j.1473-2130.2004.00115.x. [PubMed] [CrossRef] [Google Scholar]
22. Surjana D., Damian D. L. Nicotinamide in dermatology and photoprotection. Skinmed. 2011;9(6):360–365. [PubMed] [Google Scholar]
23. Williams A., Ramsden D. Nicotinamide: a double edged sword. Parkinsonism & Related Disorders. 2005;11(7):413–420. doi: 10.1016/j.parkreldis.2005.05.011. [PubMed] [CrossRef] [Google Scholar]
24. Fukushima T. Niacin metabolism and Parkinson’s disease. Environmental Health and Preventive Medicine. 2005;10(1):3–8. doi: 10.1265/ehpm.10.3. [PMC free article] [PubMed] [CrossRef] [Google Scholar]
25. Aoyama K., Matsubara K., Kondo M., et al. Nicotinamide-N-methyltransferase is higher in the lumbar cerebrospinal fluid of patients with Parkinson’s disease. Neuroscience Letters. 2001;298(1):78–80. doi: 10.1016/S0304-3940(00)01723-7. [PubMed] [CrossRef] [Google Scholar]
26. Griffin S. M., Pickard M. R., Orme R. P., Hawkins C. P., Fricker R. A. Nicotinamide promotes neuronal differentiation of mouse embryonic stem cells in vitro. Neuroreport. 2013;24(18):1041–1046. doi: 10.1097/WNR.0000000000000071. [PubMed] [CrossRef] [Google Scholar]
27. Imai S. Nicotinamide phosphoribosyltransferase (Nampt): a link between NAD biology, metabolism, and diseases. Current Pharmaceutical Design. 2009;15(1):20–28. doi: 10.2174/138161209787185814. [PMC free article] [PubMed] [CrossRef] [Google Scholar]
28. Pearl S. M., Antion M. D., Stanwood G. D., Jaumotte J. D., Kapatos G., Zigmond M. J. Effects of NADH on dopamine release in rat striatum. Synapse. 2000;36(2):95–101. doi: 10.1002/(SICI)1098-2396(200005)36:2<95::AID-SYN2>3.0.CO;2-U. [PubMed] [CrossRef] [Google Scholar]
29. Mizuno Y., Ohta S., Tanaka M., et al. Deficiencies in complex I subunits of the respiratory chain in Parkinson’s disease. Biochemical and Biophysical Research Communications. 1989;163(3):1450–1455. doi: 10.1016/0006-291X(89)91141-8. [PubMed] [CrossRef] [Google Scholar]
30. Nicklas W. J., Vyas I., Heikkila R. E. Inhibition of NADH-linked oxidation in brain mitochondria by 1-methyl-4-phenyl-pyridine, a metabolite of the neurotoxin, 1-methyl-4-phenyl-1, 2, 5, 6-tetrahydropyridine. Life Sciences. 1985;36(26):2503–2508. doi: 10.1016/0024-3205(85)90146-8. [PubMed] [CrossRef] [Google Scholar]
31. Lu L., Tang L., Wei W., et al. Nicotinamide mononucleotide improves energy activity and survival rate in an in vitro model of Parkinson’s disease. Experimental and Therapeutic Medicine. 2014;8(3):943–950. doi: 10.3892/etm.2014.1842. [PMC free article] [PubMed] [CrossRef] [Google Scholar]
32. Yang L., Ma X., He Y., et al. Sirtuin 5: a review of structure, known inhibitors and clues for developing new inhibitors. Science China Life Sciences. 2017;60(3):249–256. doi: 10.1007/s11427-016-0060-7. [PubMed] [CrossRef] [Google Scholar]
33. Liu L., Peritore C., Ginsberg J., Shih J., Arun S., Donmez G. Protective role of SIRT5 against motor deficit and dopaminergic degeneration in MPTP-induced mice model of Parkinson’s disease. Behavioural Brain Research. 2015;281:215–221. doi: 10.1016/j.bbr.2014.12.035. [PubMed] [CrossRef] [Google Scholar]
34. Fall P. A., Fredrikson M., Axelson O., Granérus A. K. Nutritional and occupational factors influencing the risk of Parkinson’s disease: a case-control study in southeastern Sweden. Movement Disorders. 1999;14(1):28–37. doi: 10.1002/1531-8257(199901)14:1<28::AID-MDS1007>3.0.CO;2-O. [PubMed] [CrossRef] [Google Scholar]
35. Hellenbrand W., Boeing H., Robra B. P., et al. Diet and Parkinson’s disease. II: a possible role for the past intake of specific nutrients. Results from a self-administered food-frequency questionnaire in a case-control study. Neurology. 1996;47(3):644–650. doi: 10.1212/WNL.47.3.644. [PubMed] [CrossRef] [Google Scholar]
36. Alisky J. M. Niacin improved rigidity and bradykinesia in a Parkinson’s disease patient but also caused unacceptable nightmares and skin rash—a case report. Nutritional Neuroscience. 2005;8(5-6):327–329. doi: 10.1080/10284150500484638. [PubMed] [CrossRef] [Google Scholar]
37. Abbott R. D., Webster Ross G., White L. R., et al. Environmental, life-style, and physical precursors of clinical Parkinson’s disease: recent findings from the Honolulu-Asia Aging Study. Journal of Neurology. 2003;250(Supplement 3):iii30–iii39. doi: 10.1007/s00415-003-1306-7. [PubMed] [CrossRef] [Google Scholar]
38. Johnson C. C., Gorell J. M., Rybicki B. A., Sanders K., Peterson E. L. Adult nutrient intake as a risk factor for Parkinson’s disease. International Journal of Epidemiology. 1999;28(6):1102–1109. doi: 10.1093/ije/28.6.1102. [PubMed] [CrossRef] [Google Scholar]