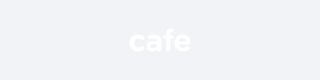
인체를 질량으로 나누면
산소 65%, 탄소 18.5%, 수소 10%, 질소 2.6%, 칼슘 1.3%, 인 0.6%, 황 0.3%, 칼륨, 나트륨, 염소 0.2%, 마그네슘 0.1%
O, C, H, N 4가지 원소가 98%를 차지한다.
이 원소들은
물 65%, 단백질 20%, 지방 12%, 무기분자 1.5%, 유기분자 0.4%의 형태로 인체에 존재한다.
https://www.youtube.com/watch?v=9StlsJBqLdY
세포단위로 살펴보면
60~100조개의 세포가 존재하는데
질량 비율로 보면 세포외기질 34.3%, 근육세포 28.6%, 지방세포 18.6%, 기타 세포 14.3%, 적혈구세포 4.2%
숫자로 보면 적혈구 세포가 85%(적혈구에는 미토콘드리아가 없음)
세포소기관의 관점으로 보면
우리 몸 건조중량의 50%가 미토콘드리아...
세포, 조직, 기관이 기능을 잘하면 우리 몸은 건강해진다.
세포기능을 잘하게 하는 한가지 방법으로 Gaseous signaling pathway가 있다.
필요조건 1. 활성산소가 많아지면 chronic inflammation pathway가 작동하여 만성염증상태의 몸이 된다.
2. 활성산소는 미토콘드리아에서 세포호흡(주로 포도당을 이용 에너지를 만드는 과정)에서 만들어진다
3. 염증은 주로 음식(food intolerance - 글루텐, 유당, 과당 intolerance), histamin intolerance, 알러지 음식에서 출발
4. 염증성 사이토카인을 지속적으로 만드는 조건은 중금속, 환경호르몬 등이 중요한 역할을 한다.
이유는 몸안의 중금속이 많은 활성산소를 만들기 때문
세포기능을 잘하게 하기 위해서는
1) 음식병원체를 차단하여 장누수를 방지. 그러면 중금속, 환경호르몬, 박테리아, 바이러스, 아플라톡신 등이 덜 유입
2) 비타민, 미네랄 결핍, 불균형은 세포대사에 문제를 일으키므로 비타민, 미네랄 문제를 해결
3) 세포(조직) 제대로 기능을 하지 못하는 세포, 세포소기관을 수리, 제거하는 역할을 하는 방법으로 self eating 자가포식이 있음
4) 건강한 세포를 더 건강하게 하는 최고의 방법은 '미토콘드리아 신생합성 운동'임.
세포소기관(특히 미토콘드리아, 리소좀, 소포체, 골지체 등)이 기능을 잘하면 세포는 스스로 건강한 딸 세포를 만들어 낸다.
건강한 세포가 많아지면 우리 몸은 스스로 건강해지는 방향으로 자리를 잡는다.
조직, 기관의 기능이 호전되면서 우리 몸은 자가치유, 자연치유의 길을 걷는다.
이렇게 세포기능을 빠르게 잘하게 하는 방법으로
고압산소, 수소수, 산화질소 등이 Gaseous signaling pathway가 있다.
구체적으로 보면
산소(고압산소, 깊은 산속 산소, 산소 가스), 수소(황화수소, 수소수), 질소(산화질소), 탄소(CO, CO2)
기체형태는 우리 몸 세포속으로 확산방식으로 전달되기 때문에 효과가 빠르고 강력하다.
산소는 고압산소치료 https://www.youtube.com/watch?v=kEdE81O-zv0
수소는 수소수 치료
https://www.youtube.com/watch?v=Ad7G3htJJPo
질소는 산화질소 형태의 치료...
Mol Pharmacol. 2019 Jul; 96(1): 109–114.
Published online 2019 Jul. doi: 10.1124/mol.118.113910
PMCID: PMC6592147
PMID: 31061006
Update on Gaseous Signaling Molecules Nitric Oxide and Hydrogen Sulfide: Strategies to Capture their Functional Activity for Human Therapeutics

and David J. Lefer
Author information Article notes Copyright and License information PMC Disclaimer
This article has been corrected. See Mol Pharmacol. 2019 August; 96(2): 127.
Abstract
Discovery of the production of gaseous molecules, such as nitric oxide and hydrogen sulfide, within the human body began a new concept in cellular signaling. Over the past 30 years, these molecules have been investigated and found to have extremely important beneficial effects in numerous chronic diseases. Gaseous signaling molecules that diffuse in three dimensions apparently contradict the selectivity and specificity afforded by normal ligand receptor binding and activation. This new concept has also created hurdles in the development of safe and efficacious drug therapy based on these molecules. Mechanisms involving formation of more stable intermediates and second messengers allow for new strategies for safe and effective delivery of these molecules for human disease. The purpose of this review is to highlight the biologic effects of nitric oxide and hydrogen sulfide, their seemingly indistinguishable effects, and how these molecules can be safely harnessed for drug development and precursors or substrates administered for human consumption through applied physiology.
인체 내에서
산화질소나
황화수소와 같은 기체 분자가 생성되는 것을 발견하면서
세포 신호에 대한 새로운 개념이 시작되었습니다.
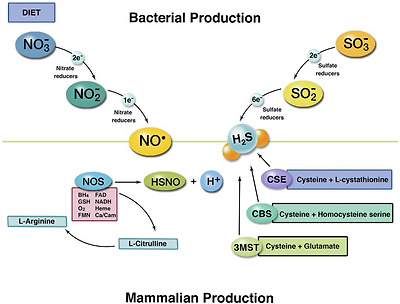
지난 30년 동안 이러한 분자들은
수많은 만성 질환에서 매우 중요한
유익한 효과를 발휘하는 것으로 밝혀졌습니다.
3차원으로 확산되는 기체 신호 분자는
정상적인 리간드 수용체 결합 및
활성화가 제공하는 선택성 및 특이성과 명백히 모순됩니다.
이러한 새로운 개념은
이러한 분자를 기반으로 한
안전하고 효과적인 약물 치료법 개발에도 장애물이 되고 있습니다.
보다 안정적인 중간체와
2차 전달체의 형성과 관련된 메커니즘을 통해
이러한 분자를 인간 질병에 안전하고 효과적으로 전달할 수 있는
새로운 전략이 가능해졌습니다.
이 리뷰의 목적은
산화질소와
황화수소의 생물학적 효과와
겉보기에는 구별하기 어려운 효과,
그리고 이러한 분자를 응용 생리학을 통해
약물 개발과 인체 섭취를 위해 투여되는 전구체 또는
기질에 안전하게 활용할 수 있는 방법을 강조하는 것입니다.
Translated with DeepL.com (free version)
Introduction
Gaseous signaling molecules, more recently termed gasotransmitters, are gaseous molecules that are either synthesized endogenously in the human body or are produced by organisms such as bacteria that live in and on the human body. These gases are then used to transmit chemical signals that induce certain and specific physiologic or biochemical changes in the human host. The notion of a gas that diffuses in three dimensions created uncertainty about how these gases could selectively and specifically activate their targets for their intended physiologic effects. The reactivity and relatively short biologic half-life of these gases are thought to limit their biologic activity. Although nitric oxide (NO) and hydrogen sulfide (H2S) have been known for many centuries, their role in human physiology is new knowledge. The fundamental and necessary nature of these molecules for normal human health is known. The loss of production of these gaseous molecules is associated with many chronic diseases that plague the health care industry, mostly involving cardiovascular disorders, including hypertension and chronic inflammation (Bryan, 2011; Polhemus and Lefer, 2014). Numerous published studies in experimental animals and even humans have revealed that delivery of bioactive NO, H2S, and/or their more stable reaction intermediates improve or prevent many of these conditions and diseases. As a result, enormous efforts have been made to develop safe and effective activators and/or stimulators of the respective enzyme systems and even donor compounds that release the specific gasotransmitter. These efforts have largely been unsuccessful. Drug discovery programs typically rely on inhibitors that limit the production of a noxious or inflammatory molecule. There are very few conditions whereby endogenous overproduction of these gaseous molecules poses a problem to human disease management. Understanding the physiologic basis for the production pathways for these molecules, both from human enzyme systems and from the microbiome, may allow for better strategies for the development of safe and effective therapies. This review highlights those production pathways for NO and H2S and reviews current and prospective technologies that may be able to harness their therapeutic activity. Signaling molecules or second messengers, by definition, should have limited half-life to avoid prolonged signal transduction. Therefore, NO and H2S share such characteristics, and strategies to produce physiologic amounts of these molecules may be necessary and sufficient to initiate and recapitulate their functions. The principle of applied physiology, rather than applied pharmacology, always prevails in terms of safety, efficacy, and limiting unwanted side effects.
가스 신호 분자는
기체 신호 전달 물질이라고도 하며,
인체에서 내인성적으로 합성되거나
인체 내부와 외부에 서식하는 박테리아와 같은 유기체에 의해 생성되는 기체 분자입니다.
이러한 가스는
숙주에게 특정 생리적 또는
생화학적 변화를 유도하는 화학적 신호를 전달하는 데 사용됩니다.
3차원으로 확산되는 가스라는 개념은
이러한 가스가 의도한 생리적 효과를 위해
표적을 선택적이고 구체적으로 활성화할 수 있는 방법에 대한
불확실성을 야기했습니다.
이러한
가스의 반응성과
상대적으로 짧은 생물학적 반감기는
생물학적 활성을 제한하는 것으로 생각됩니다.
산화질소(NO)와
황화수소(H2S)는
수세기 동안 알려져 왔지만,
인체 생리학에서 이들의 역할은 새로운 지식입니다.
정상적인 인체 건강을 위해
이러한 분자의 기본적이고 필수적인 특성은 알려져 있습니다.
이러한 기체 분자의 생산 손실은
의료 산업을 괴롭히는 많은 만성 질환과 관련이 있으며,
대부분 고혈압과
만성 염증을 포함한 심혈관 질환과 관련이 있습니다(Bryan, 2011; Polhemus and Lefer, 2014).
실험동물과 인간을 대상으로 한 수많은 연구에서
생리활성 NO,
H2S 및/또는
보다 안정적인 반응 중간체의 전달이
이러한 많은 질환과 질병을 개선하거나 예방한다는 사실이 밝혀졌습니다.
그 결과,
각 효소 시스템의 안전하고 효과적인
활성화제 및/또는 자극제,
심지어 특정 가스 전달 물질을 방출하는
기증 화합물을 개발하기 위해 막대한 노력을 기울여 왔습니다.
이러한 노력은 대부분 성공하지 못했습니다.
신약 개발 프로그램은 일반적으로
독성 또는 염증성 분자의 생성을 제한하는 억제제에 의존합니다.
이러한 기체 분자의 내인성 과잉 생산이
인간의 질병 관리에 문제를 일으키는 경우는
거의 없습니다.
인체 효소 시스템과
마이크로바이옴에서
이러한 분자의 생산 경로에 대한 생리적 근거를 이해하면
안전하고 효과적인 치료법을 개발하기 위한
더 나은 전략을 세울 수 있습니다.
이 리뷰에서는
NO와
H2S의 이러한 생산 경로를 강조하고,
이들의 치료 활성을 활용할 수 있는
현재 및 미래의 기술을 검토합니다.
신호 전달 분자 또는
2차 전달자는
장시간의 신호 전달을 피하기 위해 반감기가 제한적이어야 합니다.
따라서
NO와 H2S는 이러한 특성을 공유하며,
이러한 분자의 생리학적 양을 생산하기 위한 전략은
이들의 기능을 시작하고 요약하는 데 필요하고 충분할 수 있습니다.
응용 약리학보다는
응용 생리학의 원칙이
안전성, 효능, 원치 않는 부작용 제한 측면에서 항상 우선합니다.
Translated with DeepL.com (free version)
Translated with DeepL.com (free version)
Nitric Oxide.
Nitric oxide is now considered one of the most important signaling molecules in human and mammalian physiology. Continuous and constitutive production of NO regulates blood pressure, oxygen delivery, immune and neurocognitive function, sexual function, and many more fundamental physiologic processes. Loss of the production of NO is considered one of the earliest events in the onset and progression of most, if not all, chronic diseases (Egashira et al., 1993). Therefore, understanding how the body makes NO, what goes wrong in patients who cannot make NO, and then developing rationale therapies to restore the production of NO is considered the “Holy Grail” in medicine.
산화질소는
현재 인간과 포유류 생리학에서
가장 중요한 신호 전달 분자 중 하나로 간주됩니다.
산화질소의 지속적이고 지속적인 생성은
혈압,
산소 전달,
면역 및 신경 인지 기능,
성 기능 및 더 많은 근본적인 생리적 과정을 조절합니다.
NO 생성의 손실은
모든 만성 질환은 아니더라도
대부분의 만성 질환의 발병과 진행에서
가장 먼저 발생하는 사건 중 하나로 간주됩니다(Egashira et al., 1993).
따라서
신체가 NO를 생성하는 방법과
NO를 생성하지 못하는 환자에서
무엇이 잘못되었는지 이해한 다음
NO 생성을 회복하는 근거 요법을 개발하는 것은 의학에서 “성배”로 간주됩니다.
There are two predominant production pathways through which the human body produces NO. The first pathway to be discovered was through the five-electron oxidation of the guanidine nitrogen of l-arginine to l-citrulline with NO as a by-product (Hibbs et al., 1987). The second, more recently discovered pathway is through the serial reduction of inorganic nitrate to nitrite and to NO (Duncan et al., 1995). The first pathway is through a mammalian enzyme system known as nitric oxide synthase (NOS). The NOS enzyme requires at least half a dozen substrates and cofactors, the most important being reduced tetrahydrobiopterin (BH4). Without sufficient BH4 or when BH4 becomes oxidized, the NOS enzyme uncouples and is no longer functional (Tayeh and Marletta, 1989). Oxidation of BH4 is the rate-limiting step in NO production from NOS. The two-electron reduction of nitrate to nitrite is dependent on commensal bacteria that live in and on the human body (Lundberg et al., 2004). Mammals lack a functional nitrate reductase enzyme system, so this pathway is dependent on the proper microbiome. Once nitrite is formed, either from reduction of nitrate or from oxidation of NO, there are mammalian systems that can reduce nitrite to NO, including hemoproteins, redox active metals, mitochondria, and conditions of low pH and hypoxia (Kozlov et al., 1999; Cosby et al., 2003; Feelisch et al., 2008).
인체가
NO를 생성하는 주요 생산 경로는
두 가지가 있습니다.
첫 번째 경로는
l-아르기닌의 구아니딘 질소가 5전자 산화를 통해 부산물로
NO를 생성하여 l-시트룰린으로 전환되는 경로입니다(Hibbs 등, 1987).
최근에 발견된 두 번째 경로는
무기 질산염이 아질산염과 NO로 연속적으로
환원되는 경로입니다(Duncan et al., 1995).
첫 번째 경로는
산화질소 합성효소(NOS)로 알려진
포유류 효소 시스템을 통한 것입니다.
NOS 효소에는
최소 6가지 이상의 기질과 보조 인자가 필요한데,
가장 중요한 것은
환원된 테트라하이드로비옵테린(BH4)입니다.
BH4가 충분하지 않거나
BH4가 산화되면
NOS 효소는 결합이 해제되어
더 이상 기능하지 않습니다(Tayeh and Marletta, 1989).
BH4의 산화는
NOS에서
NO가 생성되는 속도를 제한하는 단계입니다.
질산염이 아질산염으로 2전자 환원되는 것은
인체 내부와 외부에 서식하는 공생 박테리아에 의존합니다(Lundberg et al., 2004).
포유류는
질산염 환원 효소 시스템이 없기 때문에
이 경로는 적절한 미생물 군집에 의존합니다.
질산염의 환원 또는
NO의 산화로 아질산염이 형성되면
포유류 시스템에는
혈단백질,
산화 환원 활성 금속,
미토콘드리아,
낮은 pH 및
저산소증 조건을 포함하여
아질산염을 NO로 환원할 수 있는 시스템이 있습니다(Kozlov 등, 1999; Cosby 등, 2003; Feelisch 등, 2008).
A number of different bacteria have been identified in the oral cavity that contribute to nitrate reduction and nitrite/NO formation (Doel et al., 2005; Hyde et al., 2014a,b). Eradicating oral bacteria with antiseptic mouthwash results in symptoms of NO deficiency including an increase in blood pressure (Kapil et al., 2013; Woessner et al., 2016). When NO is produced, it is thought to act primarily through binding and activation of soluble guanylyl cyclase (sGC) and production of the second messenger cyclic guanosine monophosphate (Katsuki et al., 1977). NO can also form other nitrogen oxides that can post-translationally modify critical cysteine residues on proteins affecting protein structure and function akin to phosphorylation (Lane et al., 2001). In this manner, NO regulates a host of physiologic functions.
구강 내에는
질산염 감소와 아질산염/NO 형성에 기여하는
다양한 박테리아가 존재하는 것으로 확인되었습니다(Doel et al., 2005; Hyde et al., 2014a,b).
살균 구강 청결제로
구강 내 박테리아를 박멸하면
혈압 상승을 포함한 NO 결핍 증상이 나타납니다(Kapil et al., 2013; Woessner et al., 2016).
NO가 생성되면
주로 가용성 구아닐 시클라제(sGC)의 결합 및 활성화와
두 번째 메신저인 사이클릭 구아노신 모노포스페이트의 생성을 통해
작용하는 것으로 생각됩니다(Katsuki et al., 1977).
NO는 또한
인산화와 유사하게
단백질 구조와 기능에 영향을 미치는
단백질의 중요한 시스테인 잔기를 번역 후 변형할 수 있는
다른 질소 산화물을 형성할 수 있습니다(Lane et al., 2001).
이러한 방식으로
NO는
다양한 생리적 기능을 조절합니다.
The fundamental question then becomes how to restore or recapitulate NO-based signaling. Understanding both pathways for NO production allows one to focus on restoring these pathways, including strategies to preserve BH4 redox status to maintain NOS coupling and function, as well as restoring the microbiome in the oral cavity, in the intestines, and on the skin. Nitrite prevents BH4 oxidation and maintains NOS function (Stokes et al., 2009). Nitrite production in the oral cavity resulting from reduction of nitrate by the oral nitrate-reducing bacteria is one way of restoring NO. This method requires sufficient nitrate from the diet and the right oral bacteria. This pathway becomes disrupted when humans do not consume enough nitrate from vegetables and when the oral microbiome is disrupted by antiseptic mouthwash or antibiotic use (Bryan, 2018). Providing nitrite in the form of therapy is a logical, safe, and effective strategy for restoring NO-based signaling. In fact, nitrite has even been shown to be the endocrine mediator of NO-based signaling (Elrod et al., 2008).
그렇다면
근본적인 질문은
NO 기반 신호를 어떻게 복원하거나
요약할 것인가 하는 것입니다.
NO 생성의 두 가지 경로를 이해하면
구강, 장, 피부의 마이크로바이옴을 복원할 뿐만 아니라
NOS 결합과 기능을 유지하기 위해
BH4 산화 환원 상태를 보존하는 전략을 포함하여
이러한 경로를 복원하는 데 집중할 수 있습니다.
아질산염은
BH4 산화를 방지하고
NOS 기능을 유지합니다(Stokes et al., 2009).
구강 내 질산염 환원 박테리아에 의한
질산염 환원으로 인한
구강 내 아질산염 생성은
NO를 회복하는 한 가지 방법입니다.
이 방법을 사용하려면
식단에서 충분한 질산염과
적절한 구강 박테리아가 필요합니다.
이 경로는
사람이 채소에서 충분한 질산염을 섭취하지 않거나
살균성 구강 청결제 또는
항생제 사용으로 구강 미생물이 파괴되면 중단됩니다(Bryan, 2018).
아질산염을
치료의 형태로 제공하는 것은
NO 기반 신호를 회복하기 위한
논리적이고 안전하며 효과적인 전략입니다.
실제로
아질산염은
NO 기반 신호의 내분비 매개체라는 사실도 밝혀졌습니다(Elrod et al., 2008).
Many studies have been published that describe demonstrating the safety and efficacy of nitrite in humans within a large range of doses. Capsules containing sodium nitrite at doses of 160 and 320 mg have been used to investigate toxicity and pharmacokinetics. Nitrite, even at high doses of 320 mg, did not show any clinical toxicity as measured by methemoglobinemia (<15%) (Greenway et al., 2012). Therapy using a 80-mg nitrite capsule caused a statistically significant drop in systolic blood pressure with no changes on diastolic blood pressures. Longer-term studies using 80–160 mg of nitrite capsules in a randomized, placebo control, double-blind study over 10 weeks led to an increase in plasma nitrite both acutely and chronically and was well tolerated (DeVan et al., 2016). Endothelial function and carotid artery elasticity significantly improved (DeVan et al., 2016). Other studies have shown significant improvements in measures of motor and cognitive outcomes in healthy middle-aged and older adults using 80 and 160 mg of nitrite (62 ± 7 years) (Justice et al., 2015). Unlike nitrate, the effects of nitrite are not dependent on or require the present of oral nitrate- reducing bacteria. Doses of nitrite appear to be safe even at doses that far exceed daily human consumption.
다양한 용량 범위에서
아질산염의 인체 내 안전성과 효능을 입증하는
많은 연구가 발표되었습니다.
독성 및 약동학을 조사하기 위해
아질산나트륨을 160mg 및 320mg 용량으로 함유한
캡슐이 사용되었습니다.
아질산염은
320mg의 고용량에서도
메트헤모글로빈혈증(<15%)으로 측정한 임상 독성을 나타내지 않았습니다(Greenway et al., 2012).
80mg 아질산염 캡슐을 사용한 치료는
수축기 혈압을 통계적으로 유의하게 떨어뜨리고
이완기 혈압에는 변화를 일으키지 않았습니다.
10주 동안 무작위, 위약 대조, 이중 맹검 연구에서
아질산염 캡슐 80-160mg을 사용한 장기 연구에서
급성 및 만성적으로 혈장 아질산염이 증가했으며
내약성이 우수했습니다(DeVan et al., 2016).
내피 기능과 경동맥 탄력이 유의미하게 개선되었습니다(DeVan 외., 2016).
다른 연구에서는
건강한 중년 및 노년층(62 ± 7세)을 대상으로
아질산염 80mg과 160mg을 섭취한 결과
운동 및 인지 기능 측정에서 유의미한 개선이 나타났습니다(Justice et al., 2015).
질산염과 달리 아질산염의 효과는
구강 질산염 환원 박테리아의 존재 여부에 의존하거나 필요하지 않습니다.
아질산염은
사람의 일일 섭취량을 훨씬 초과하는 용량에서도 안전한 것으로 보입니다.
The amounts of nitrite administered in the aforementioned studies are much higher than one would normally consume in an ordinary diet. This is because nitrite is inefficiently reduced to NO at physiologic concentrations of oxygen (Bryan et al., 2005; Feelisch et al., 2008). Therefore, more is needed to get any appreciable amount of NO produced, especially in people who are NO-deficient. We have discovered natural product chemistry that can reduce nitrite to NO even in the presence of physiologic amounts of oxygen, thereby providing an exogenous source of NO in vivo (Tang et al., 2009). The premise of this technology is that if your body cannot make NO owing to endothelial dysfunction, lack of oral nitrate-reducing bacteria, use of antiseptic mouthwash or proton pump inhibitors (PPIs), then this therapy will provide an exogenous source of NO. These discoveries have been used to develop a commericial product (Neo40; HumanN, Inc) which uses 15–20 mg of supplemental sodium nitrite to account for differences in endogenous production, along with the natural nitrite reductase in the form of an orally disintegrating tablet. Studies have shown that it could improve cardiovascular risk factors in older patients, significantly reduce triglycerides, and reduce blood pressure (Zand et al., 2011).
앞서 언급한 연구에서
투여된 아질산염의 양은
일반적인 식단에서 일반적으로 섭취하는 것보다 훨씬 더 많은 양입니다.
이는 아질산염이 생리적 산소 농도에서
NO로 비효율적으로 환원되기 때문입니다(Bryan 외., 2005; Feelisch 외., 2008).
따라서,
특히 NO가 결핍된 사람들에게서
상당한 양의 NO를 생성하려면 더 많은 양이 필요합니다.
우리는 생리학적 양의 산소가 있는 상태에서도
아질산염을 NO로 환원하여
생체 내 외인성 NO 공급원을 제공할 수 있는
천연물 화학 물질을 발견했습니다(Tang et al., 2009).
이 기술의 전제는
내피 기능 장애,
구강 질산염 감소 박테리아 부족,
살균 구강 세정제 또는
양성자 펌프 억제제(PPI) 사용으로 인해
신체가 NO를 만들 수 없는 경우
이 요법이 외인성 NO 공급원을 제공한다는 것입니다.
이러한 발견은
내인성 생성의 차이를 설명하기 위해
15~20mg의 아질산나트륨 보충제와
구강 붕해 정제 형태의 천연 아질산염 환원효소를 사용하는
상업용 제품(Neo40; HumanN, Inc)을 개발하는 데 사용되었습니다.
연구에 따르면 이 제품은
고령 환자의 심혈관 위험 요인을 개선하고
중성지방을 크게 낮추며 혈압을 낮출 수 있는 것으로 나타났습니다(Zand et al., 2011).
Single and acute administration of this lozenge leads to peak plasma levels of nitrite around 1.5 µM after 20 minutes. In pediatric patients with a condition called argininosuccinic aciduria (ASA), which is an inborn error in metabolism that causes hyperammonemia along with hypertension, coagulopathy, renal and liver dysfunction, the nitrite lozenge, led to a significant reduction in blood pressure when multiple classes of antihypertensive medications were ineffective. The lozenge also improved renal function and cognition and reversed cardiac hypertrophy (Nagamani et al., 2012). In another randomized, controlled study using the nitrite lozenge showed a significantly reduction in blood pressure, a significant increase in blood vessel dilation, and a significant improvement in endothelial function and arterial compliance (Houston and Hays, 2014). Furthermore, in a study of prehypertensive patients (blood pressure > 120/80 < 139/89), administration of one lozenge twice daily for 30 days lowered both systolic and diastolic blood pressure levels by 12 and 6 mm Hg, respectively (Biswas et al., 2015), along with improvements in functional capacity as measured by a 6-minute walk test. In an exercise study, the nitrite lozenge significantly improved exercise performance (Lee et al., 2015). Most recently, in subjects with stable carotid plaque, the NO lozenge led to a 11% reduction in carotid plaque as measured by carotid intima media thickness (CIMT) after 6 months (Lee, 2016).
이 로젠지를 단회 급성 투여하면
20분 후 아질산염의 혈장 최고 수치가
약 1.5 µM에 도달합니다.
고혈압,
응고 병증,
신장 및 간 기능 장애와 함께
고암모니아혈증을 유발하는 선천성 대사 장애인
아르기니노숙신산뇨증(ASA)이라는 질환이 있는
소아 환자에서 아질산염 로젠지는
여러 종류의 항고혈압제가 효과가 없을 때
혈압을 현저히 낮추는 것으로 나타났습니다.
또한 이 사탕은
신장 기능과 인지능력을 개선하고 심장 비대를 역전시켰습니다(나가마니 외., 2012).
아질산염 로젠지를 사용한 또 다른 무작위 대조 연구에서는 혈압이 유의하게 감소하고 혈관 확장이 유의하게 증가했으며 내피 기능과 동맥 순응도가 유의하게 개선된 것으로 나타났습니다(Houston and Hays, 2014). 또한 고혈압 전단계 환자(혈압 > 120/80 < 139/89)를 대상으로 한 연구에서 30일 동안 하루 두 번 로젠지 1정을 투여한 결과 수축기 혈압과 이완기 혈압이 각각 12mmHg와 6mmHg 낮아졌으며, 6분 걷기 테스트를 통해 측정한 기능 능력이 개선되었습니다(Biswas et al., 2015). 한 운동 연구에서 아질산염 사탕은 운동 능력을 크게 향상시켰습니다(Lee et al., 2015). 가장 최근에는 경동맥 플라크가 안정된 피험자에게 NO 로젠지를 투여한 결과, 6개월 후 경동맥 내막 두께(CIMT)로 측정한 경동맥 플라크가 11% 감소한 것으로 나타났습니다(Lee, 2016).
To put this in perspective, treatment with statins (mean treatment duration of 25.6 months) reveals regression and slowing of progression of CIMT of approximately 2.7% (−0.04) after motr than 2 years (Bedi et al., 2010). Using the nitric oxide lozenge, CIMT decreased an average of 0.073 mm or 10.9% after 6 months (Lee, 2016). Similarly, this same patented technology in the form of a concentrated beet root powder (Superbeets; HumanN, Inc.) has been shown to attenuate peripheral chemoreflex sensitivity without a concomitant change in spontaneous cardiovagal baroreflex sensitivity. The concentrated beet powder also reduces systemic blood pressure and mean arterial blood pressure in older adults (Bock et al., 2018). These studies clearly demonstrate the safety and efficacy of low supplemental doses of nitrite. Furthermore, providing an exogenous source of NO in humans appears to correct for any deficiencies from dietary exposure, pharmacologic inhibition by antiseptics, or PPIs.
Other studies in mice reveal that simply giving nitrate or nitrite in the drinking water can alter the oral microbiome to allow for colonization of more nitrate-reducing bacteria (Hyde et al., 2014b). In this manner, nitrite therapy may provide the basis for restoring eNOS function and restoring the proper NO-generating bacteria in the oral cavity.
스타틴 치료(평균 치료 기간 25.6개월)는
2년 이상 치료 후 약 2.7%(-0.04)의
CIMT 퇴행 및 진행 둔화 효과를 나타냅니다(Bedi et al., 2010).
산화질소 로젠지를 사용하면
6개월 후 CIMT가 평균 0.073mm 또는 10.9% 감소했습니다(Lee, 2016).
이와 유사하게,
농축 비트 뿌리 분말 형태의 동일한 특허 기술(슈퍼비트, 휴먼앤)은
자발적 심혈관 압력반사 민감도의 수반되는 변화 없이
말초 체모반사 민감도를 약화시키는 것으로 나타났습니다(휴먼앤, Inc.).
또한 농축 비트 분말은
노인의 전신 혈압과 평균 동맥 혈압을 낮추는 것으로 나타났습니다(Bock et al., 2018).
이러한 연구는
아질산염의 저용량 보충제의 안전성과 효능을 명확하게 입증합니다.
또한,
사람에게 외인성 아질산염 공급원을 제공하면
식이 노출, 방부제에 의한 약리학적 억제 또는
PPI로 인한 결핍을 보완할 수 있는 것으로 보입니다.
생쥐를 대상으로 한 다른 연구에서는
단순히 식수에 질산염이나 아질산염을 투여하는 것만으로도
구강 미생물 군집을 변화시켜 질산염을 줄이는
박테리아가 더 많이 서식할 수 있다는 사실이 밝혀졌습니다(Hyde et al., 2014b).
이러한 방식으로
아질산염 요법은
eNOS 기능을 회복하고
구강 내 적절한 NO 생성 박테리아를 회복하는 기초를 제공할 수 있습니다.
Hydrogen Sulfide.
Hydrogen sulfide (H2S) is a naturally occurring compound that is produced throughout the human body. Just like NO, H2S is acutely toxic at high concentrations This toxicity is attributed to inhibition of mitochondrial cytochrome c oxidase, carbonic anhydrase, monoamine oxidase, Na+/K+-ATPase, and cholinesterases (Beauchamp et al., 1984). H2S also reacts with the oxy forms of myoglobin and hemoglobin (FeII-O2) generating the sulfheme derivative, thereby reducing oxygen binding and transport (Pietri et al., 2011). The modern history of H2S is mostly associated with its toxic effects. It was only recently that H2S may serve as an important biologic mediator (Abe and Kimura, 1996). Since then, studies have shown that H2S is involved in a number of biologic signaling mechanisms in numerous biologic systems. Similar to sGC, as a target for NO-based signaling, Na/K-ATPase is a known physiologic target for H2S (Xia et al., 2009). Also, similar to post-translational modification of critical cysteine residues by nitrosation of NO-based signaling, H2S can lead to persulfidation, thereby affecting the structure and function of proteins (Paul and Snyder, 2015). Among other biologic effects, H2S has been reported to have antihypertensive and cytoprotective properties (Benavides et al., 2007; Polhemus and Lefer, 2014), again like NO. Most of the research on H2S has focused mainly on the enzymatic production in the heart, kidneys, vasculature, and brain. The biologic action of H2S produced by the gut microbiota has been recently examined. Given the prominent role of H2S in numerous diseases, many therapeutic targets have been discovered for H2S therapy. The molecular targets of H2S include proteins, enzymes, transcription factors, and membrane ion channels and other proteins. Cysteine is the major source and substrate of H2S in mammals. H2S is catalyzed by three enzymes: cystathionine β-synthase (CBS), cystathionine γ-lyase (CSE), and 3-mercaptopyruvate sulfurtransferase (3-MST). The enzyme 3-MST is localized in mitochondria and CBS and CSE exist in the cytosol (Polhemus and Lefer, 2014).
Like in NO production, commensal bacteria provide the human host with a source of hydrogen sulfide. Sulfate-reducing bacteria (SRB) are ubiquitous and common to the mammalian colon (Tomasova et al., 2016b). The dominant genera are Desulfovibrio (D. piger, D. desulfuricans), Desulfobacter, Desulfobulbus and Desulfotomaculum (Rabus et al., 2015). There are two substrates that are essential for SRB to produce H2S. Those are any form of sulfate and an electron donor for the sulfate reduction. A sulfate-rich diet has been shown to result in the increased growth of D. piger and increased H2S production in the colon of humans and mice (Gibson et al., 1988; Rey et al., 2013). D. piger can also use sulfated glycans. Sulfate-reducing bacteria therefore are a common source of H2S in the gut of mammals. Several anaerobic bacterial strains (Escherichia coli, Salmonella enterica, Clostridia, and Enterobacter aerogenes) convert cysteine to H2S, pyruvate and ammonia by cysteine desulfhydrase (Kumagai et al., 1975; Awano et al., 2005). In addition, gut bacteria can also produce H2S by sulfite reduction. Sulfite reductase activity is present in many species of bacteria, such as E. coli, Salmonella, Enterobacter, Klebsiella, Bacillus, Staphylococcus, Corynebacterium, and Rhodococcus spp. (Blachier et al., 2010).
The total sulfide concentration produced in the large intestine has been quantified to be in the range of 0.2–3.4 mmol/liter in humans; however, since the feces of humans and rodents have a large binding capacity for sulfur, it is estimated that less than 8% of total sulfide is in a free form (Jørgensen and Mortensen, 2001; Levitt et al., 2002). Colonic epithelial cells are more efficient in converting sulfide into thiosulfate than other tissues (Furne et al., 2001). Infusion of radioactive H2S into the cecum of rats showed that all the absorbed H2S from the infusion was immediately oxidized to thiosulfate (Levitt et al., 1999). Flannigan et al. (2011) reported that fecal samples of germ-free mice contained 50% less H2S compared with feces of controls. Germ-free mice have significantly less free H2S in plasma and gastrointestinal tissues compared with control mice. CSE activity in tissue of germ-free mice is significantly reduced, whereas tissue cysteine levels appear to be significantly elevated compared with conventional mice. These data suggest that the microbiota profoundly regulates systemic bioavailability and metabolism of H2S (Shen et al., 2013). Eliminated vitamin B6, a CSE and CBS cofactor, in the diet causes a 50% reduction of fecal H2S, likely owing to the reduction of enzymatic H2S synthesis in colonic tissues. Interestingly, after 6 weeks of the vitamin B6-deficient diet, the fecal H2S levels in mice were restored to normal, suggesting that the H2S generation in the gut of germ-free mice was shifted toward microbial production pathways by increasing the SRB activity (Flannigan et al., 2011).
Although vitamin B6 deficiency is relatively rare, many people may still be deficient despite consuming the recommended daily allowance of vitamin B6 (Morris et al., 2008). Rats treated with neomycin show significantly lower levels of thiosulfate and sulfane sulfur in the portal vein blood, but not in peripheral blood (Tomasova et al., 2016a). Overproduction of H2S in the colon has been implicated in colonic inflammation and cancer (Roediger et al., 1993; Cao et al., 2010); however, other studies suggest that colonic epithelial cells are well adapted to the H2S-rich environment, and that H2S plays a beneficial role in the protection of the gut brain barrier (Goubern et al., 2007; Wallace, 2010; Motta et al., 2015). H2S may serve as an energy source for colonic epithelial cells as a result of ATP formation from H2S oxidation (Goubern et al., 2007). H2S also promotes colonic mucus production, thereby maintaining the integrity of bacterial biofilms (Motta et al., 2015). H2S protects and reverses damage induced by chronic administration of nonsteroidal anti-inflammatory drugs (Blackler et al., 2015).
The main dietary sources of exposure to sulfur compounds in the human diet are inorganic sulfates in drinking water and sulfur-containing amino acids in proteins derived from plants and animals. Only two of the 20 amino acids are sulfur-containing amino acids: methionine and cysteine. Methionine is unable to be synthesized by the human body and must be consumed through the diet. Dietary methionine intake can increase cysteine levels. Cysteine is known as a semiessential amino acid since humans can produce it endogenously from methionine; however, the function of the enzymes required for the production of cysteine from methionine declines with age (Koc and Gladyshev, 2007); therefore, with increasing age, less cysteine is produced endogenously. Excess consumption of cysteine and methionine from the diet is converted and stored as glutathione. Cysteine availability is the rate-limiting factor for glutathione biosynthesis from glutamate, glycine, and cysteine.
황화수소(H2S)는
인체 전체에서 생성되는 자연 발생 화합물입니다.
NO와 마찬가지로
H2S는 고농도에서
급성 독성을 나타냅니다.
이러한 독성은
미토콘드리아 시토크롬 c 산화효소,
탄산탈수효소,
모노아민 산화효소,
Na+/K+-ATPase 및
콜린 에스 테라제의 억제에 기인합니다(Beauchamp et al., 1984).
또한 H2S는
산소 형태의 미오글로빈 및 헤모글로빈(FeII-O2)과 반응하여
황화합물 유도체를 생성하여
산소 결합 및 수송을 감소시킵니다(Pietri et al., 2011).
H2S의 현대 역사는
대부분 독성 효과와 관련이 있습니다.
H2S가 중요한 생물학적 매개체 역할을 할 수 있다는 사실이 밝혀진 것은
최근의 일입니다(Abe and Kimura, 1996).
그 이후로 여러 연구에 따르면
H2S는 수많은 생물학적 시스템에서
여러 생물학적 신호 전달 메커니즘에 관여하는 것으로
밝혀졌습니다.
NO 기반 신호 전달의 표적으로서
sGC와 유사하게
Na/K-ATPase는 H2S의 생리학적 표적으로 알려져 있습니다(Xia et al., 2009).
또한,
NO 기반 신호의 질소화에 의한
중요한 시스테인 잔기의 번역 후 변형과 유사하게
H2S는 과황화를 유발하여
단백질의 구조와 기능에 영향을 미칠 수 있습니다(Paul and Snyder, 2015).
다른 생물학적 효과 중에서도
H2S는 NO와 마찬가지로
항고혈압 및 세포 보호 특성을 가진 것으로 보고되었습니다(Benavides et al., 2007; Polhemus and Lefer, 2014).
H2S에 대한 대부분의 연구는
주로 심장, 신장, 혈관계 및 뇌의 효소 생성에 중점을 두었습니다.
최근 장내 미생물에 의해 생성되는
H2S의 생물학적 작용이 조사되고 있습니다.
수많은 질병에서 H2S의 두드러진 역할을 고려할 때,
H2S 치료를 위한 많은 치료 표적이 발견되었습니다.
H2S의 분자 표적에는
단백질, 효소, 전사인자, 막 이온 채널 및 기타 단백질이 포함됩니다.
시스테인은
포유류에서 H2S의 주요 공급원이자 기질입니다.
H2S는
시스타티오닌 β-신타제(CBS),
시스타티오닌 γ-리아제(CSE),
3-메르캅토피루베이트 설퍼트랜스퍼라제(3-MST) 등
세 가지 효소에 의해 촉매 작용을 합니다.
3-MST 효소는
미토콘드리아에 국한되어 있고
CBS와 CSE는 세포질에 존재합니다(Polhemus and Lefer, 2014).
NO 생산과 마찬가지로 공생 박테리아는
인간 숙주에게 황화수소의 공급원을 제공합니다.
황산염 환원 박테리아(SRB)는
포유류 결장에 흔하게 존재하며 어디에나 존재합니다(Tomasova et al., 2016b).
우세한 속은
데설포비브리오(D. 피거, D. 데설퓨리칸스),
데설포박터, 데설포불버스, 데설포토마쿨룸입니다(Rabus et al., 2015).
SRB가 H2S를 생산하는 데
필수적인 두 가지 기질이 있습니다.
모든 형태의 황산염과
황산염 환원을 위한 전자 공여체가 그것입니다.
황산염이 풍부한 식단은
사람과 쥐의 결장에서 D. piger의 성장을 증가시키고
H2S 생성을 증가시키는 것으로 나타났습니다(Gibson et al., 1988; Rey et al., 2013).
D. 피거는 또한
황산화된 글리칸을 사용할 수 있습니다.
따라서 황산염 환원 박테리아는
포유류의 장내에서 H2S의 일반적인 공급원입니다.
여러 혐기성 박테리아 균주(대장균, 살모넬라 엔테리카, 클로스트리디아, 엔테로박터 에어로제네스)는
시스테인 탈황수소효소에 의해 시스테인을
H2S, 피루베이트 및 암모니아로 전환합니다(쿠마가이 등, 1975; 아와노 등, 2005).
또한 장내 박테리아는
아황산염 환원을 통해
H2S를 생성할 수도 있습니다.
아황산염 환원효소 활성은
대장균, 살모넬라, 엔테로박터, 클렙시엘라, 바실러스, 포도상구균, 코리네박테리움, 로도코커스 등 많은 박테리아 종에 존재합니다(Blachier et al., 2010).
대장에서 생성되는 총 황화물 농도는
사람의 경우 0.2-3.4 mmol/리터 범위로 정량화되었지만,
사람과 설치류의 대변은 황에 대한 결합력이 크기 때문에 총 황화물의 8% 미만이 유리 형태로 존재하는 것으로 추정됩니다(Jørgensen and Mortensen, 2001; Levitt et al., 2002).
대장 상피 세포는
다른 조직보다 황화물을 티오황산염으로 전환하는 데 더 효율적입니다(Furne et al., 2001).
방사성 H2S를 쥐의 맹장에 주입하면 주입에서 흡수된 모든 H2S가 즉시 티오황산염으로 산화되는 것으로 나타났습니다(Levitt et al., 1999). Flannigan 등(2011)은 세균이 없는 쥐의 분변 샘플에 대조군 쥐의 분변에 비해 H2S가 50% 더 적게 함유되어 있다고 보고했습니다. 세균이 없는 마우스는 대조군 마우스에 비해 혈장 및 위장 조직에서 유리 H2S가 현저히 적습니다. 세균이 없는 마우스의 조직에서 CSE 활성은 현저히 감소한 반면, 조직 시스테인 수치는 일반 마우스에 비해 현저히 상승한 것으로 나타났습니다. 이러한 데이터는 미생물총이 H2S의 전신 생체이용률과 대사를 크게 조절한다는 것을 시사합니다(Shen et al., 2013). 식이에서 CSE 및 CBS 보조 인자인 비타민 B6를 제거하면 분변의 H2S가 50% 감소하는데, 이는 대장 조직에서 효소적 H2S 합성이 감소하기 때문일 가능성이 높습니다. 흥미롭게도 비타민 B6 결핍 식이 6주 후 생쥐의 분변 H2S 수치가 정상으로 회복되었으며, 이는 세균이 없는 생쥐의 장에서 H2S 생성이 SRB 활성을 증가시켜 미생물 생산 경로로 이동했음을 시사합니다(Flannigan et al., 2011).
비타민 B6 결핍은
비교적 드물지만,
많은 사람이 일일 권장량을 섭취함에도 불구하고
여전히 비타민 B6가 결핍되어 있을 수 있습니다(Morris et al., 2008).
네오마이신으로 치료한 쥐는 문맥혈에서 티오설페이트와 설판황 수치가 현저히 낮았지만 말초혈에서는 그렇지 않았습니다(Tomasova 등, 2016a). 대장 내 H2S의 과잉 생산은 대장 염증 및 암과 관련이 있는 것으로 알려져 있지만(Roediger 외, 1993; Cao 외, 2010), 다른 연구에 따르면 대장 상피 세포는 H2S가 풍부한 환경에 잘 적응하며, H2S가 장 뇌 장벽 보호에 유익한 역할을 한다고 합니다(Goubern 외, 2007; Wallace, 2010; Motta 외, 2015). H2S는 H2S 산화에 의한 ATP 형성의 결과로 대장 상피 세포의 에너지원으로 작용할 수 있습니다(Goubern et al., 2007). 또한 H2S는 대장 점액 생성을 촉진하여 박테리아 바이오필름의 무결성을 유지합니다(Motta et al., 2015). H2S는 비스테로이드성 항염증제의 만성 투여로 인해 유발된 손상을 보호하고 역전시킵니다(Blackler et al., 2015).
인간 식단에서 황 화합물에 노출되는 주요 식이 공급원은 식수의 무기 황산염과 식물과 동물에서 추출한 단백질의 황 함유 아미노산입니다. 20가지 아미노산 중 메티오닌과 시스테인 두 가지만이 황 함유 아미노산입니다. 메티오닌은 인체에서 합성할 수 없으므로 반드시 식단을 통해 섭취해야 합니다. 식이 메티오닌 섭취는 시스테인 수치를 높일 수 있습니다. 시스테인은 메티오닌으로부터 내인성적으로 생산할 수 있기 때문에 준 필수 아미노산으로 알려져 있지만, 메티오닌으로부터 시스테인을 생산하는 데 필요한 효소의 기능은 나이가 들어감에 따라 감소하므로(Koc and Gladyshev, 2007), 나이가 증가하면 내인성적으로 생산되는 시스테인 양이 줄어듭니다. 식단에서 시스테인과 메티오닌을 과도하게 섭취하면 글루타티온으로 전환되어 저장됩니다. 시스테인 가용성은 글루타메이트, 글리신, 시스테인으로부터 글루타치온 생합성 속도를 제한하는 요소입니다.
You Cannot Have One without the Other.
Growing evidence shows that there is “crosstalk” between sulfide and NO. NO and H2S elicit many of the same physiologic actions in the cardiovascular system, including: vasodilation, regulation of mitochondrial respiration, proangiogenic effects, inhibition of apoptosis, and antioxidant effects. Although H2S and NO modulate independent signaling cascades, there is now strong evidence that these gaseous signaling molecules operate in a cooperative fashion to modulate a multitude of important actions (Cortese-Krott et al., 2015). eNOS function is regulated tightly by post-translational modifications (i.e., phosphorylation of amino acids Ser-1177 and Thr-495) that enhance or inhibit NO production by eNOS (Dimmeler et al., 1999). In a pressure-overload murine heart failure, Kondo et al. (2013) reported that mice treated with an H2S donor (i.e., SG1002) were protected against adverse cardiac remodeling and heart failure. Interestingly, the authors also reported a significant increase in phosphorylation of the eNOS activation site, Ser-1177, in mice treated with SG1002 compared with the control group. This increase in eNOS phosphorylation was accompanied by increased NO production, as demonstrated by increased circulating and tissue levels of NO metabolites. The authors suggest that the protective actions of H2S donor therapy in heart failure is mediated in part via increased myocardial and circulating NO levels.
Furthermore, Predmore et al. (2012) investigated the cardioprotective effects of the garlic-derived H2S donor diallyl trisulfide in a murine model of myocardial ischemia and reperfusion. The authors reported that treatment with diallyl trisulfide significantly reduced the area of myocardial necrosis concomitant with marked increases in plasma nitrite, nitrate, and nitrosylated protein levels 30 minutes after injection. These data provide additional support for the concept of H2S-NO cooperativity and clearly demonstrate that administration of exogenous H2S by the use of H2S donors activates eNOS to augment NO bioavailability and protect the heart and circulation against cardiovascular diseases.
A recent study by King et al. (2014) investigated the physiologic regulation of eNOS-NO mediated cell signaling by endogenous H2S generated from cystathionine γ lyase (CSE) by using a CSE mutant mouse model. Interestingly, King et al. (2014) reported that basal circulating and myocardial tissue H2S and NO levels were significantly decreased in CSE knockout mice compared with wild-type mice. In this same study, myocardial oxidative stress and eNOS uncoupling were significantly increased in the CSE knockout mouse. The authors treated the CSE-deficient mice with H2S donor therapy for 7 days and reported significant increases in circulating and tissue levels of NO that were similar to those of wild-type animals. Finally, the authors evaluated the effects of H2S donors on myocardial ischemia/reperfusion injury in both eNOS knockout and eNOS phosphomutants. The cardioprotective actions were completely abrogated in mice deficient in eNOS or mice with dysfunctional eNOS. These data very strongly suggest that endogenous H2S derived from the H2S-producing enzyme CSE attenuates endothelial oxidative stress, resulting in improved eNOS activation status and the production of physiologically relevant NO levels. Thus, H2S acts as a chaperone to preserve eNOS-NO signaling and normal cardiovascular homeostasis. Since sulfide is a strong nucleophile, it is also possible that the effects of hydrogen sulfide may be through protection of BH4 oxidation and maintaining the proper redox status for optimal NOS production of NO. Another potential mechanism is through H2S-mediated S-sulfhydration of eNOS, which promotes phosphorylation, thereby inhibiting its S-nitrosylation and increasing eNOS dimerization with the consequential improved NO production (Altaany et al., 2014) or a combination of all the above.
황화물과 NO 사이에 "누화"가 있다는 증거가 점점 더 많아지고 있습니다. NO와 H2S는 혈관 확장, 미토콘드리아 호흡 조절, 혈관 신생 효과, 세포 사멸 억제, 항산화 효과 등 심혈관계에서 동일한 생리적 작용을 많이 유도합니다. H2S와 NO는 독립적인 신호 캐스케이드를 조절하지만, 현재 이러한 가스 신호 분자가 협력적인 방식으로 작동하여 여러 가지 중요한 작용을 조절한다는 강력한 증거가 있습니다(Cortese-Krott et al., 2015). eNOS 기능은 번역 후 변형(즉, 아미노산 Ser-1177 및 Thr-495의 인산화)에 의해 엄격하게 조절되어 eNOS의 NO 생산을 강화하거나 억제합니다(Dimmeler et al., 1999). 압력 과부하 쥐 심부전 실험에서 콘도 등(2013)은 H2S 기증자(즉, SG1002)로 치료한 쥐가 불리한 심장 리모델링과 심부전으로부터 보호되었다고 보고했습니다. 흥미롭게도 저자들은 SG1002로 치료한 마우스에서 대조군에 비해 eNOS 활성화 부위인 Ser-1177의 인산화가 현저히 증가했다고 보고했습니다. 이러한 eNOS 인산화 증가는 NO 대사 산물의 순환 및 조직 수준 증가로 입증된 바와 같이 NO 생성 증가를 동반했습니다. 저자들은 심부전에서 H2S 기증자 치료의 보호 작용이 부분적으로 심근 및 순환 NO 수치 증가를 통해 매개된다고 제안합니다.
또한 프레드모어 외(2012)는 심근 허혈 및 재관류의 쥐 모델에서 마늘 유래 H2S 공여자 디알릴 트리설파이드의 심장 보호 효과를 조사했습니다. 저자들은 디알릴 트리설파이드 치료가 주사 30분 후 혈장 아질산염, 질산염, 니트로실화 단백질 수치의 현저한 증가와 함께 심근 괴사 면적을 현저히 감소시켰다고 보고했습니다. 이러한 데이터는 H2S-NO 협력성의 개념을 추가로 뒷받침하며, H2S 기증자를 사용하여 외인성 H2S를 투여하면 eNOS가 활성화되어 NO 생체 이용률을 높이고 심혈관 질환으로부터 심장과 순환을 보호한다는 사실을 명확하게 보여줍니다.
King 등(2014)의 최근 연구에서는 CSE 돌연변이 마우스 모델을 사용하여 시스타티오닌 γ 리아제(CSE)에서 생성된 내인성 H2S에 의한 eNOS-NO 매개 세포 신호의 생리적 조절을 조사했습니다. 흥미롭게도 King 등(2014)은 야생형 마우스에 비해 CSE 녹아웃 마우스에서 기저 순환 및 심근 조직 H2S와 NO 수치가 현저히 감소했다고 보고했습니다. 같은 연구에서 CSE 녹아웃 마우스에서 심근 산화 스트레스와 eNOS 결합 해제가 유의하게 증가했습니다. 저자들은 CSE 결핍 마우스를 7일 동안 H2S 공여 요법으로 치료한 결과, 야생형 동물과 유사한 순환 및 조직 수준의 NO가 유의미하게 증가했다고 보고했습니다. 마지막으로 저자들은 eNOS 녹아웃 및 eNOS 인산화 변이체 모두에서 심근 허혈/재관류 손상에 대한 H2S 공여체의 효과를 평가했습니다. 심장 보호 작용은 eNOS가 결핍된 마우스 또는 기능 장애가 있는 eNOS 마우스에서 완전히 사라졌습니다. 이러한 데이터는 H2S 생성 효소 CSE에서 유래한 내인성 H2S가 내피 산화 스트레스를 약화시켜 eNOS 활성화 상태를 개선하고 생리적으로 관련된 NO 수준을 생성한다는 것을 매우 강력하게 시사합니다. 따라서 H2S는 eNOS-NO 신호와 정상적인 심혈관 항상성을 보존하는 보호자 역할을 합니다. 황화물은 강력한 핵친화성이기 때문에 황화수소의 효과는 BH4 산화를 보호하고 최적의 NO 생산을 위한 적절한 산화 환원 상태를 유지함으로써 나타날 수도 있습니다. 또 다른 잠재적 메커니즘은 인산화를 촉진하여 eNOS의 S-니트로실화를 억제하고 결과적으로 NO 생성을 개선하여 eNOS 이량체화를 증가시키는 H2S 매개 S-황수화를 통한 것입니다(Altaany et al., 2014) 또는 위의 모든 조합을 통해 이루어질 수 있습니다.
Conclusions
Despite these two gaseous signaling molecules being known to be cytoprotective and necessary for health and disease prevention, drug development around NO and H2S-active therapies has been slow and unsuccessful. Perhaps the best approach to restoring production of these and other gaseous signals is to provide the body what it needs to produce these naturally and allow endogenous systems and nature to do their job. This will require at least three considerations: 1) optimizing the enzyme systems that produce both NO and H2S by providing the essential cofactors and substrates from the diet for enzymatic production (i.e., tetrahydrobiopterin, vitamin B6, glutathione, and such for proper nutrition); 2) restoring normal microbiota and flora that are part of the nitrate- and sulfate-reducing bacteria (modifying diet, probiotics, prebiotics); 3) reducing the use of antibiotics, antiseptic mouthwash, nonsteroidal anti-inflammatory drugs, PPIs, and such that interfere with NO and H2S production. Common practices such as rinsing with antiseptic mouthwash and overuse of antibiotics disrupt the bacterial production of NO and H2S. Diets that do not include sufficient nitrate and sulfate from foods will disrupt their production. This concept is illustrated in Fig. 1. Therapies or strategies that provide the human body with an exogenous source of NO or H2S may also be a viable approach. An NO-generating lozenge has been shown to recapitulate NO-based signaling in humans (Zand et al., 2011; Nagamani et al., 2012; Houston and Hays, 2014; Biswas et al., 2015; Lee et al., 2015; Lee, 2016), possibly owing to known endocrine or hormone-like effects of NO (Elrod et al., 2008). It is unclear at this time whether providing hydrogen sulfide gas directly to humans will have similar effects. The science clearly shows that providing nitrate or nitrite or sodium sulfide in the form of supplementation can restore and recapitulate NO- and H2S-based signaling, which provides an optimal opportunity to direct therapies of applied physiology.
이 두 가지 기체 신호 분자는 세포를 보호하고 건강과 질병 예방에 필요한 것으로 알려져 있지만, NO와 H2S 활성 요법에 대한 약물 개발은 더디고 성공적이지 못했습니다. 아마도 이러한 기체 신호와 기타 기체 신호의 생성을 회복하는 가장 좋은 방법은 신체가 자연적으로 생성하는 데 필요한 것을 제공하고 내인성 시스템과 자연이 그 일을 하도록 하는 것입니다. 이를 위해서는 최소한 세 가지를 고려해야 합니다: 1) 효소 생산을 위한 필수 보조인자와 기질을 식단에서 제공함으로써 NO와 H2S를 모두 생산하는 효소 시스템을 최적화하는 것(예 테트라하이드로비옵테린, 비타민 B6, 글루타치온 등 적절한 영양 공급), 2) 질산염 및 황산염을 줄이는 박테리아의 일부인 정상적인 미생물과 식물상 복원(식단 수정, 프로바이오틱스, 프리바이오틱스), 3) NO 및 H2S 생성을 방해하는 항생제, 소독용 구강청결제, 비스테로이드성 항염증제, PPI 등의 사용을 줄이세요. 소독용 구강 청결제로 헹구거나 항생제를 남용하는 것과 같은 일반적인 관행은 박테리아의 NO 및 H2S 생성을 방해합니다. 음식에서 충분한 질산염과 황산염을 포함하지 않는 식단은 이들 물질의 생성을 방해합니다. 이 개념은 그림 1에 설명되어 있습니다. 인체에 외인성 NO 또는 H2S 공급원을 제공하는 요법이나 전략도 실행 가능한 접근법일 수 있습니다. NO 생성 마름모꼴 사탕은 인간에서 NO 기반 신호를 요약하는 것으로 나타났습니다
(Zand 외, 2011; Nagamani 외, 2012; Houston and Hays, 2014; Biswas 외, 2015; Lee 외, 2015; Lee, 2016), 아마도 NO의 알려진 내분비 또는 호르몬 유사 효과(Elrod 외, 2008)로 인한 것일 수 있습니다. 황화수소 가스를 인간에게 직접 공급하는 것이 유사한 효과를 가져올지는 현재로서는 불분명합니다. 과학은 질산염이나 아질산염 또는 황화나트륨을 보충제 형태로 제공하면 NO 및 H2S 기반 신호를 복원하고 요약하여 응용 생리학의 직접적인 치료를 위한 최적의 기회를 제공할 수 있다는 것을 분명히 보여줍니다.
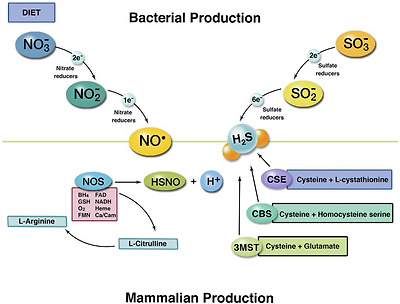
The two pathways for production of NO and H2S in humans. The top left section illustrates commensal bacterial production of NO from nitrate-reducing bacteria. The top right depicts H2S production from sulfate-reducing bacteria. Bottom left illustrates the mechanism of eNOS production of NO and the required substrates and cofactors. Bottom right shows the three known enzymatic pathways for H2S production. Once produced, the two molecules can react to form thionitrite to potentiate NO-based signaling. Furthermore, H2S can protect from BH4 oxidation and/or S-sulfhydration of cysteine residues on eNOS to improve production of NO.
Acknowledgments
N.S.B. is a founder and shareholder, HumanN, Inc.; a consultant and shareholder, SAJE Pharma; and receives royalties from patents from the University of Texas Health Science Center at Houston.
Abbreviations
3-MST | 3-mercaptopyruvate sulfurtransferase |
ASA | argininosuccinic aciduria |
BH4 | tetrahydrobiopterin |
CA | carbonic anhydrase |
CBS | cystathionine β-synthase |
CIMT | carotid intima media thickness |
CSE | cystathionine γ-lyase |
H2S | hydrogen sulfide |
NO | nitric oxide |
NOS | nitric oxide synthase |
PPI | proton pump inhibitor |
sGC | soluble guanylyl cyclase |
SRB | sulfate-reducing bacteria |
Authorship Contributions
Wrote or contributed to the writing of the manuscript: Bryan, Lefer.
Footnotes
https://doi.org/10.1124/mol.118.113910.
References
- Abe K, Kimura H. (1996) The possible role of hydrogen sulfide as an endogenous neuromodulator. J Neurosci 16:1066–1071. [PMC free article] [PubMed] [Google Scholar]
- Altaany Z, Ju Y, Yang G, Wang R. (2014) The coordination of S-sulfhydration, S-nitrosylation, and phosphorylation of endothelial nitric oxide synthase by hydrogen sulfide. Sci Signal 7:ra87. [PubMed] [Google Scholar]
- Awano N, Wada M, Mori H, Nakamori S, Takagi H. (2005) Identification and functional analysis of Escherichia coli cysteine desulfhydrases. Appl Environ Microbiol 71:4149–4152. [PMC free article] [PubMed] [Google Scholar]
- Beauchamp RO, Jr, Bus JS, Popp JA, Boreiko CJ, Andjelkovich DA. (1984) A critical review of the literature on hydrogen sulfide toxicity. Crit Rev Toxicol 13:25–97. [PubMed] [Google Scholar]
- Bedi US, Singh M, Singh PP, Bhuriya R, Bahekar A, Molnar J, Khosla S, Arora R. (2010) Effects of statins on progression of carotid atherosclerosis as measured by carotid intimal—medial thickness: a meta-analysis of randomized controlled trials. J Cardiovasc Pharmacol Ther 15:268–273. [PubMed] [Google Scholar]
- Benavides GA, Squadrito GL, Mills RW, Patel HD, Isbell TS, Patel RP, Darley-Usmar VM, Doeller JE, Kraus DW. (2007) Hydrogen sulfide mediates the vasoactivity of garlic. Proc Natl Acad Sci USA 104:17977–17982. [PMC free article] [PubMed] [Google Scholar]
- Biswas OS, Gonzalez VR, Schwarz ER. (2015) Effects of an oral nitric oxide supplement on functional capacity and blood pressure in adults with prehypertension. J Cardiovasc Pharmacol Ther 20:52–58. [PubMed] [Google Scholar]
- Blachier F, Davila AM, Mimoun S, Benetti PH, Atanasiu C, Andriamihaja M, Benamouzig R, Bouillaud F, Tomé D. (2010) Luminal sulfide and large intestine mucosa: friend or foe? Amino Acids 39:335–347. [PubMed] [Google Scholar]
- Blackler RW, Motta JP, Manko A, Workentine M, Bercik P, Surette MG, Wallace JL. (2015) Hydrogen sulphide protects against NSAID-enteropathy through modulation of bile and the microbiota. Br J Pharmacol 172:992–1004. [PMC free article] [PubMed] [Google Scholar]
- Bock JM, Ueda K, Schneider AC, Hughes WE, Limberg JK, Bryan NS, Casey DP. (2018) Inorganic nitrate supplementation attenuates peripheral chemoreflex sensitivity but does not improve cardiovagal baroreflex sensitivity in older adults. Am J Physiol Heart Circ Physiol 314:H45–H51. [PMC free article] [PubMed] [Google Scholar]
- Bryan NS. (2011) Application of nitric oxide in drug discovery and development. Expert Opin Drug Discov 6:1139–1154. [PubMed] [Google Scholar]
- Bryan NS. (2018) Functional nitric oxide nutrition to combat cardiovascular disease. Curr Atheroscler Rep 20:21. [PubMed] [Google Scholar]
- Bryan NS, Fernandez BO, Bauer SM, Garcia-Saura MF, Milsom AB, Rassaf T, Maloney RE, Bharti A, Rodriguez J, Feelisch M. (2005) Nitrite is a signaling molecule and regulator of gene expression in mammalian tissues. Nat Chem Biol 1:290–297. [PubMed] [Google Scholar]
- Cao Q, Zhang L, Yang G, Xu C, Wang R. (2010) Butyrate-stimulated H2S production in colon cancer cells. Antioxid Redox Signal 12:1101–1109. [PubMed] [Google Scholar]
- Cortese-Krott MM, Fernandez BO, Kelm M, Butler AR, Feelisch M. (2015) On the chemical biology of the nitrite/sulfide interaction. Nitric Oxide 46:14–24. [PubMed] [Google Scholar]
- Cosby K, Partovi KS, Crawford JH, Patel RP, Reiter CD, Martyr S, Yang BK, Waclawiw MA, Zalos G, Xu X, et al. (2003) Nitrite reduction to nitric oxide by deoxyhemoglobin vasodilates the human circulation. Nat Med 9:1498–1505. [PubMed] [Google Scholar]
- DeVan AE, Johnson LC, Brooks FA, Evans TD, Justice JN, Cruickshank-Quinn C, Reisdorph N, Bryan NS, McQueen MB, Santos-Parker JR, et al. (2016) Effects of sodium nitrite supplementation on vascular function and related small metabolite signatures in middle-aged and older adults. J Appl Physiol (1985) 120:416–425. [PMC free article] [PubMed] [Google Scholar]
- Dimmeler S, Fleming I, Fisslthaler B, Hermann C, Busse R, Zeiher AM. (1999) Activation of nitric oxide synthase in endothelial cells by Akt-dependent phosphorylation. Nature 399:601–605. [PubMed] [Google Scholar]
- Doel JJ, Benjamin N, Hector MP, Rogers M, Allaker RP. (2005) Evaluation of bacterial nitrate reduction in the human oral cavity. Eur J Oral Sci 113:14–19. [PubMed] [Google Scholar]
- Duncan C, Dougall H, Johnston P, Green S, Brogan R, Leifert C, Smith L, Golden M, Benjamin N. (1995) Chemical generation of nitric oxide in the mouth from the enterosalivary circulation of dietary nitrate. Nat Med 1:546–551. [PubMed] [Google Scholar]
- Egashira K, Inou T, Hirooka Y, Kai H, Sugimachi M, Suzuki S, Kuga T, Urabe Y, Takeshita A. (1993) Effects of age on endothelium-dependent vasodilation of resistance coronary artery by acetylcholine in humans. Circulation 88:77–81. [PubMed] [Google Scholar]
- Elrod JW, Calvert JW, Gundewar S, Bryan NS, Lefer DJ. (2008) Nitric oxide promotes distant organ protection: evidence for an endocrine role of nitric oxide. Proc Natl Acad Sci USA 105:11430–11435. [PMC free article] [PubMed] [Google Scholar]
- Feelisch M, Fernandez BO, Bryan NS, Garcia-Saura MF, Bauer S, Whitlock DR, Ford PC, Janero DR, Rodriguez J, Ashrafian H. (2008) Tissue processing of nitrite in hypoxia: an intricate interplay of nitric oxide-generating and -scavenging systems. J Biol Chem 283:33927–33934. [PMC free article] [PubMed] [Google Scholar]
- Flannigan KL, McCoy KD, Wallace JL. (2011) Eukaryotic and prokaryotic contributions to colonic hydrogen sulfide synthesis. Am J Physiol Gastrointest Liver Physiol 301:G188–G193. [PubMed] [Google Scholar]
- Furne J, Springfield J, Koenig T, DeMaster E, Levitt MD. (2001) Oxidation of hydrogen sulfide and methanethiol to thiosulfate by rat tissues: a specialized function of the colonic mucosa. Biochem Pharmacol 62:255–259. [PubMed] [Google Scholar]
- Gibson GR, Macfarlane GT, Cummings JH. (1988) Occurrence of sulphate-reducing bacteria in human faeces and the relationship of dissimilatory sulphate reduction to methanogenesis in the large gut. J Appl Bacteriol 65:103–111. [PubMed] [Google Scholar]
- Goubern M, Andriamihaja M, Nübel T, Blachier F, Bouillaud F. (2007) Sulfide, the first inorganic substrate for human cells. FASEB J 21:1699–1706. [PubMed] [Google Scholar]
- Greenway FL, Predmore BL, Flanagan DR, Giordano T, Qiu Y, Brandon A, Lefer DJ, Patel RP, Kevil CG. (2012) Single-dose pharmacokinetics of different oral sodium nitrite formulations in diabetes patients. Diabetes Technol Ther 14:552–560. [PMC free article] [PubMed] [Google Scholar]
- Hibbs JB, Jr, Taintor RR, Vavrin Z. (1987) Macrophage cytotoxicity: role for L-arginine deiminase and imino nitrogen oxidation to nitrite. Science 235:473–476. [PubMed] [Google Scholar]
- Houston M, Hays L. (2014) Acute effects of an oral nitric oxide supplement on blood pressure, endothelial function, and vascular compliance in hypertensive patients. J Clin Hypertens (Greenwich) 16:524–529. [PMC free article] [PubMed] [Google Scholar]
- Hyde ER, Andrade F, Vaksman Z, Parthasarathy K, Jiang H, Parthasarathy DK, Torregrossa AC, Tribble G, Kaplan HB, Petrosino JF, et al. (2014a) Metagenomic analysis of nitrate-reducing bacteria in the oral cavity: implications for nitric oxide homeostasis. PLoS One 9:e88645. [PMC free article] [PubMed] [Google Scholar]
- Hyde ER, Luk B, Cron S, Kusic L, McCue T, Bauch T, Kaplan H, Tribble G, Petrosino JF, Bryan NS. (2014b) Characterization of the rat oral microbiome and the effects of dietary nitrate. Free Radic Biol Med 77:249–257. [PubMed] [Google Scholar]
- Jørgensen J, Mortensen PB. (2001) Hydrogen sulfide and colonic epithelial metabolism: implications for ulcerative colitis. Dig Dis Sci 46:1722–1732. [PubMed] [Google Scholar]
- Justice JN, Johnson LC, DeVan AE, Cruickshank-Quinn C, Reisdorph N, Bassett CJ, Evans TD, Brooks FA, Bryan NS, Chonchol MB, et al. (2015) Improved motor and cognitive performance with sodium nitrite supplementation is related to small metabolite signatures: a pilot trial in middle-aged and older adults. Aging (Albany NY) 7:1004–1021. [PMC free article] [PubMed] [Google Scholar]
- Kapil V, Haydar SM, Pearl V, Lundberg JO, Weitzberg E, Ahluwalia A. (2013) Physiological role for nitrate-reducing oral bacteria in blood pressure control. Free Radic Biol Med 55:93–100. [PMC free article] [PubMed] [Google Scholar]
- Katsuki S, Arnold W, Mittal C, Murad F. (1977) Stimulation of guanylate cyclase by sodium nitroprusside, nitroglycerin and nitric oxide in various tissue preparations and comparison to the effects of sodium azide and hydroxylamine. J Cyclic Nucleotide Res 3:23–35. [PubMed] [Google Scholar]
- King AL, Polhemus DJ, Bhushan S, Otsuka H, Kondo K, Nicholson CK, Bradley JM, Islam KN, Calvert JW, Tao YX, et al. (2014) Hydrogen sulfide cytoprotective signaling is endothelial nitric oxide synthase-nitric oxide dependent. Proc Natl Acad Sci USA 111:3182–3187. [PMC free article] [PubMed] [Google Scholar]
- Koc A, Gladyshev VN. (2007) Methionine sulfoxide reduction and the aging process. Ann N Y Acad Sci 1100:383–386. [PubMed] [Google Scholar]
- Kondo K, Bhushan S, King AL, Prabhu SD, Hamid T, Koenig S, Murohara T, Predmore BL, Gojon G, Sr, Gojon G, Jr, et al. (2013) H2S protects against pressure overload-induced heart failure via upregulation of endothelial nitric oxide synthase. Circulation 127:1116–1127. [PMC free article] [PubMed] [Google Scholar]
- Kozlov AV, Staniek K, Nohl H. (1999) Nitrite reductase activity is a novel function of mammalian mitochondria. FEBS Lett 454:127–130. [PubMed] [Google Scholar]
- Kumagai H, Sejima S, Choi Y, Tanaka H, Yamada H. (1975) Crystallization and properties of cysteine desulfhydrase from Aerobacter aerogenes. FEBS Lett 52:304–307. [PubMed] [Google Scholar]
- Lane P, Hao G, Gross SS. (2001) S-nitrosylation is emerging as a specific and fundamental posttranslational protein modification: head-to-head comparison with O-phosphorylation. Sci STKE 2001:re1. [PubMed] [Google Scholar]
- Lee E. (2016) Effects of nitric oxide on carotid intima media thickness: a pilot study. Altern Ther Health Med 22(Suppl 2):32–34. [PubMed] [Google Scholar]
- Lee J, Kim HT, Solares GJ, Kim K, Ding Z, Ivy JL. (2015) Caffeinated nitric oxide-releasing lozenge improves cycling time trial performance. Int J Sports Med 36:107–112. [PubMed] [Google Scholar]
- Levitt MD, Furne J, Springfield J, Suarez F, DeMaster E. (1999) Detoxification of hydrogen sulfide and methanethiol in the cecal mucosa. J Clin Invest 104:1107–1114. [PMC free article] [PubMed] [Google Scholar]
- Levitt MD, Springfield J, Furne J, Koenig T, Suarez FL. (2002) Physiology of sulfide in the rat colon: use of bismuth to assess colonic sulfide production. J Appl Physiol (1985) 92:1655–1660. [PubMed] [Google Scholar]
- Lundberg JO, Weitzberg E, Cole JA, Benjamin N. (2004) Nitrate, bacteria and human health. Nat Rev Microbiol 2:593–602. [PubMed] [Google Scholar]
- Morris MS, Picciano MF, Jacques PF, Selhub J. (2008) Plasma pyridoxal 5′-phosphate in the US population: the National Health and Nutrition Examination Survey, 2003-2004. Am J Clin Nutr 87:1446–1454. [PubMed] [Google Scholar]
- Motta JP, Flannigan KL, Agbor TA, Beatty JK, Blackler RW, Workentine ML, Da Silva GJ, Wang R, Buret AG, Wallace JL. (2015) Hydrogen sulfide protects from colitis and restores intestinal microbiota biofilm and mucus production. Inflamm Bowel Dis 21:1006–1017. [PubMed] [Google Scholar]
- Nagamani SC, Campeau PM, Shchelochkov OA, Premkumar MH, Guse K, Brunetti-Pierri N, Chen Y, Sun Q, Tang Y, Palmer D, et al. (2012) Nitric-oxide supplementation for treatment of long-term complications in argininosuccinic aciduria. Am J Hum Genet 90:836–846. [PMC free article] [PubMed] [Google Scholar]
- Paul BD, Snyder SH. (2015) H2S: a novel gasotransmitter that signals by sulfhydration. Trends Biochem Sci 40:687–700. [PMC free article] [PubMed] [Google Scholar]
- Pietri R, Román-Morales E, López-Garriga J. (2011) Hydrogen sulfide and hemeproteins: knowledge and mysteries. Antioxid Redox Signal 15:393–404. [PMC free article] [PubMed] [Google Scholar]
- Polhemus DJ, Lefer DJ. (2014) Emergence of hydrogen sulfide as an endogenous gaseous signaling molecule in cardiovascular disease. Circ Res 114:730–737. [PMC free article] [PubMed] [Google Scholar]
- Predmore BL, Kondo K, Bhushan S, Zlatopolsky MA, King AL, Aragon JP, Grinsfelder DB, Condit ME, Lefer DJ. (2012) The polysulfide diallyl trisulfide protects the ischemic myocardium by preservation of endogenous hydrogen sulfide and increasing nitric oxide bioavailability. Am J Physiol Heart Circ Physiol 302:H2410–H2418. [PMC free article] [PubMed] [Google Scholar]
- Rabus R, Venceslau SS, Wöhlbrand L, Voordouw G, Wall JD, Pereira IA. (2015) A post-genomic view of the ecophysiology, catabolism and biotechnological relevance of sulphate-reducing prokaryotes. Adv Microb Physiol 66:55–321. [PubMed] [Google Scholar]
- Rey FE, Gonzalez MD, Cheng J, Wu M, Ahern PP, Gordon JI. (2013) Metabolic niche of a prominent sulfate-reducing human gut bacterium. Proc Natl Acad Sci USA 110:13582–13587. [PMC free article] [PubMed] [Google Scholar]
- Roediger WEW, Duncan A, Kapaniris O, Millard S. (1993) Sulphide impairment of substrate oxidation in rat colonocytes: a biochemical basis for ulcerative colitis? Clin Sci (Lond) 85:623–627. [PubMed] [Google Scholar]
- Shen X, Carlström M, Borniquel S, Jädert C, Kevil CG, Lundberg JO. (2013) Microbial regulation of host hydrogen sulfide bioavailability and metabolism. Free Radic Biol Med 60:195–200. [PMC free article] [PubMed] [Google Scholar]
- Stokes KY, Dugas TR, Tang Y, Garg H, Guidry E, Bryan NS. (2009) Dietary nitrite prevents hypercholesterolemic microvascular inflammation and reverses endothelial dysfunction. Am J Physiol Heart Circ Physiol 296:H1281–H1288. [PubMed] [Google Scholar]
- Tang Y, Garg H, Geng YJ, Bryan NS. (2009) Nitric oxide bioactivity of traditional Chinese medicines used for cardiovascular indications. Free Radic Biol Med 47:835–840. [PubMed] [Google Scholar]
- Tayeh MA, Marletta MA. (1989) Macrophage oxidation of L-arginine to nitric oxide, nitrite, and nitrate: tetrahydrobiopterin is required as a cofactor. J Biol Chem 264:19654–19658. [PubMed] [Google Scholar]
- Tomasova L, Dobrowolski L, Jurkowska H, Wróbel M, Huc T, Ondrias K, Ostaszewski R, Ufnal M. (2016a) Intracolonic hydrogen sulfide lowers blood pressure in rats. Nitric Oxide 60:50–58. [PubMed] [Google Scholar]
- Tomasova L, Konopelski P, Ufnal M. (2016b) Gut bacteria and hydrogen sulfide: the new old players in circulatory system homeostasis. Molecules 21:1–18. [PMC free article] [PubMed] [Google Scholar]
- Wallace JL. (2010) Physiological and pathophysiological roles of hydrogen sulfide in the gastrointestinal tract. Antioxid Redox Signal 12:1125–1133. [PubMed] [Google Scholar]
- Woessner M, Smoliga JM, Tarzia B, Stabler T, Van Bruggen M, Allen JD. (2016) A stepwise reduction in plasma and salivary nitrite with increasing strengths of mouthwash following a dietary nitrate load. Nitric Oxide 54:1–7. [PubMed] [Google Scholar]
- Xia M, Chen L, Muh RW, Li PL, Li N. (2009) Production and actions of hydrogen sulfide, a novel gaseous bioactive substance, in the kidneys. J Pharmacol Exp Ther 329:1056–1062. [PMC free article] [PubMed] [Google Scholar]
- Zand J, Lanza F, Garg HK, Bryan NS. (2011) All-natural nitrite and nitrate containing dietary supplement promotes nitric oxide production and reduces triglycerides in humans. Nutr Res 31:262–269. [PubMed] [Google Scholar]
- Abe K, Kimura H. (1996) The possible role of hydrogen sulfide as an endogenous neuromodulator. J Neurosci 16:1066–1071. [PMC free article] [PubMed] [Google Scholar]
- Altaany Z, Ju Y, Yang G, Wang R. (2014) The coordination of S-sulfhydration, S-nitrosylation, and phosphorylation of endothelial nitric oxide synthase by hydrogen sulfide. Sci Signal 7:ra87. [PubMed] [Google Scholar]
- Awano N, Wada M, Mori H, Nakamori S, Takagi H. (2005) Identification and functional analysis of Escherichia coli cysteine desulfhydrases. Appl Environ Microbiol 71:4149–4152. [PMC free article] [PubMed] [Google Scholar]
- Beauchamp RO, Jr, Bus JS, Popp JA, Boreiko CJ, Andjelkovich DA. (1984) A critical review of the literature on hydrogen sulfide toxicity. Crit Rev Toxicol 13:25–97. [PubMed] [Google Scholar]
- Bedi US, Singh M, Singh PP, Bhuriya R, Bahekar A, Molnar J, Khosla S, Arora R. (2010) Effects of statins on progression of carotid atherosclerosis as measured by carotid intimal—medial thickness: a meta-analysis of randomized controlled trials. J Cardiovasc Pharmacol Ther 15:268–273. [PubMed] [Google Scholar]
- Benavides GA, Squadrito GL, Mills RW, Patel HD, Isbell TS, Patel RP, Darley-Usmar VM, Doeller JE, Kraus DW. (2007) Hydrogen sulfide mediates the vasoactivity of garlic. Proc Natl Acad Sci USA 104:17977–17982. [PMC free article] [PubMed] [Google Scholar]
- Biswas OS, Gonzalez VR, Schwarz ER. (2015) Effects of an oral nitric oxide supplement on functional capacity and blood pressure in adults with prehypertension. J Cardiovasc Pharmacol Ther 20:52–58. [PubMed] [Google Scholar]
- Blachier F, Davila AM, Mimoun S, Benetti PH, Atanasiu C, Andriamihaja M, Benamouzig R, Bouillaud F, Tomé D. (2010) Luminal sulfide and large intestine mucosa: friend or foe? Amino Acids 39:335–347. [PubMed] [Google Scholar]
- Blackler RW, Motta JP, Manko A, Workentine M, Bercik P, Surette MG, Wallace JL. (2015) Hydrogen sulphide protects against NSAID-enteropathy through modulation of bile and the microbiota. Br J Pharmacol 172:992–1004. [PMC free article] [PubMed] [Google Scholar]
- Bock JM, Ueda K, Schneider AC, Hughes WE, Limberg JK, Bryan NS, Casey DP. (2018) Inorganic nitrate supplementation attenuates peripheral chemoreflex sensitivity but does not improve cardiovagal baroreflex sensitivity in older adults. Am J Physiol Heart Circ Physiol 314:H45–H51. [PMC free article] [PubMed] [Google Scholar]
- Bryan NS. (2011) Application of nitric oxide in drug discovery and development. Expert Opin Drug Discov 6:1139–1154. [PubMed] [Google Scholar]
- Bryan NS. (2018) Functional nitric oxide nutrition to combat cardiovascular disease. Curr Atheroscler Rep 20:21. [PubMed] [Google Scholar]
- Bryan NS, Fernandez BO, Bauer SM, Garcia-Saura MF, Milsom AB, Rassaf T, Maloney RE, Bharti A, Rodriguez J, Feelisch M. (2005) Nitrite is a signaling molecule and regulator of gene expression in mammalian tissues. Nat Chem Biol 1:290–297. [PubMed] [Google Scholar]
- Cao Q, Zhang L, Yang G, Xu C, Wang R. (2010) Butyrate-stimulated H2S production in colon cancer cells. Antioxid Redox Signal 12:1101–1109. [PubMed] [Google Scholar]
- Cortese-Krott MM, Fernandez BO, Kelm M, Butler AR, Feelisch M. (2015) On the chemical biology of the nitrite/sulfide interaction. Nitric Oxide 46:14–24. [PubMed] [Google Scholar]
- Cosby K, Partovi KS, Crawford JH, Patel RP, Reiter CD, Martyr S, Yang BK, Waclawiw MA, Zalos G, Xu X, et al. (2003) Nitrite reduction to nitric oxide by deoxyhemoglobin vasodilates the human circulation. Nat Med 9:1498–1505. [PubMed] [Google Scholar]
- DeVan AE, Johnson LC, Brooks FA, Evans TD, Justice JN, Cruickshank-Quinn C, Reisdorph N, Bryan NS, McQueen MB, Santos-Parker JR, et al. (2016) Effects of sodium nitrite supplementation on vascular function and related small metabolite signatures in middle-aged and older adults. J Appl Physiol (1985) 120:416–425. [PMC free article] [PubMed] [Google Scholar]
- Dimmeler S, Fleming I, Fisslthaler B, Hermann C, Busse R, Zeiher AM. (1999) Activation of nitric oxide synthase in endothelial cells by Akt-dependent phosphorylation. Nature 399:601–605. [PubMed] [Google Scholar]
- Doel JJ, Benjamin N, Hector MP, Rogers M, Allaker RP. (2005) Evaluation of bacterial nitrate reduction in the human oral cavity. Eur J Oral Sci 113:14–19. [PubMed] [Google Scholar]
- Duncan C, Dougall H, Johnston P, Green S, Brogan R, Leifert C, Smith L, Golden M, Benjamin N. (1995) Chemical generation of nitric oxide in the mouth from the enterosalivary circulation of dietary nitrate. Nat Med 1:546–551. [PubMed] [Google Scholar]
- Egashira K, Inou T, Hirooka Y, Kai H, Sugimachi M, Suzuki S, Kuga T, Urabe Y, Takeshita A. (1993) Effects of age on endothelium-dependent vasodilation of resistance coronary artery by acetylcholine in humans. Circulation 88:77–81. [PubMed] [Google Scholar]
- Elrod JW, Calvert JW, Gundewar S, Bryan NS, Lefer DJ. (2008) Nitric oxide promotes distant organ protection: evidence for an endocrine role of nitric oxide. Proc Natl Acad Sci USA 105:11430–11435. [PMC free article] [PubMed] [Google Scholar]
- Feelisch M, Fernandez BO, Bryan NS, Garcia-Saura MF, Bauer S, Whitlock DR, Ford PC, Janero DR, Rodriguez J, Ashrafian H. (2008) Tissue processing of nitrite in hypoxia: an intricate interplay of nitric oxide-generating and -scavenging systems. J Biol Chem 283:33927–33934. [PMC free article] [PubMed] [Google Scholar]
- Flannigan KL, McCoy KD, Wallace JL. (2011) Eukaryotic and prokaryotic contributions to colonic hydrogen sulfide synthesis. Am J Physiol Gastrointest Liver Physiol 301:G188–G193. [PubMed] [Google Scholar]
- Furne J, Springfield J, Koenig T, DeMaster E, Levitt MD. (2001) Oxidation of hydrogen sulfide and methanethiol to thiosulfate by rat tissues: a specialized function of the colonic mucosa. Biochem Pharmacol 62:255–259. [PubMed] [Google Scholar]
- Gibson GR, Macfarlane GT, Cummings JH. (1988) Occurrence of sulphate-reducing bacteria in human faeces and the relationship of dissimilatory sulphate reduction to methanogenesis in the large gut. J Appl Bacteriol 65:103–111. [PubMed] [Google Scholar]
- Goubern M, Andriamihaja M, Nübel T, Blachier F, Bouillaud F. (2007) Sulfide, the first inorganic substrate for human cells. FASEB J 21:1699–1706. [PubMed] [Google Scholar]
- Greenway FL, Predmore BL, Flanagan DR, Giordano T, Qiu Y, Brandon A, Lefer DJ, Patel RP, Kevil CG. (2012) Single-dose pharmacokinetics of different oral sodium nitrite formulations in diabetes patients. Diabetes Technol Ther 14:552–560. [PMC free article] [PubMed] [Google Scholar]
- Hibbs JB, Jr, Taintor RR, Vavrin Z. (1987) Macrophage cytotoxicity: role for L-arginine deiminase and imino nitrogen oxidation to nitrite. Science 235:473–476. [PubMed] [Google Scholar]
- Houston M, Hays L. (2014) Acute effects of an oral nitric oxide supplement on blood pressure, endothelial function, and vascular compliance in hypertensive patients. J Clin Hypertens (Greenwich) 16:524–529. [PMC free article] [PubMed] [Google Scholar]
- Hyde ER, Andrade F, Vaksman Z, Parthasarathy K, Jiang H, Parthasarathy DK, Torregrossa AC, Tribble G, Kaplan HB, Petrosino JF, et al. (2014a) Metagenomic analysis of nitrate-reducing bacteria in the oral cavity: implications for nitric oxide homeostasis. PLoS One 9:e88645. [PMC free article] [PubMed] [Google Scholar]
- Hyde ER, Luk B, Cron S, Kusic L, McCue T, Bauch T, Kaplan H, Tribble G, Petrosino JF, Bryan NS. (2014b) Characterization of the rat oral microbiome and the effects of dietary nitrate. Free Radic Biol Med 77:249–257. [PubMed] [Google Scholar]
- Jørgensen J, Mortensen PB. (2001) Hydrogen sulfide and colonic epithelial metabolism: implications for ulcerative colitis. Dig Dis Sci 46:1722–1732. [PubMed] [Google Scholar]
- Justice JN, Johnson LC, DeVan AE, Cruickshank-Quinn C, Reisdorph N, Bassett CJ, Evans TD, Brooks FA, Bryan NS, Chonchol MB, et al. (2015) Improved motor and cognitive performance with sodium nitrite supplementation is related to small metabolite signatures: a pilot trial in middle-aged and older adults. Aging (Albany NY) 7:1004–1021. [PMC free article] [PubMed] [Google Scholar]
- Kapil V, Haydar SM, Pearl V, Lundberg JO, Weitzberg E, Ahluwalia A. (2013) Physiological role for nitrate-reducing oral bacteria in blood pressure control. Free Radic Biol Med 55:93–100. [PMC free article] [PubMed] [Google Scholar]
- Katsuki S, Arnold W, Mittal C, Murad F. (1977) Stimulation of guanylate cyclase by sodium nitroprusside, nitroglycerin and nitric oxide in various tissue preparations and comparison to the effects of sodium azide and hydroxylamine. J Cyclic Nucleotide Res 3:23–35. [PubMed] [Google Scholar]
- King AL, Polhemus DJ, Bhushan S, Otsuka H, Kondo K, Nicholson CK, Bradley JM, Islam KN, Calvert JW, Tao YX, et al. (2014) Hydrogen sulfide cytoprotective signaling is endothelial nitric oxide synthase-nitric oxide dependent. Proc Natl Acad Sci USA 111:3182–3187. [PMC free article] [PubMed] [Google Scholar]
- Koc A, Gladyshev VN. (2007) Methionine sulfoxide reduction and the aging process. Ann N Y Acad Sci 1100:383–386. [PubMed] [Google Scholar]
- Kondo K, Bhushan S, King AL, Prabhu SD, Hamid T, Koenig S, Murohara T, Predmore BL, Gojon G, Sr, Gojon G, Jr, et al. (2013) H2S protects against pressure overload-induced heart failure via upregulation of endothelial nitric oxide synthase. Circulation 127:1116–1127. [PMC free article] [PubMed] [Google Scholar]
- Kozlov AV, Staniek K, Nohl H. (1999) Nitrite reductase activity is a novel function of mammalian mitochondria. FEBS Lett 454:127–130. [PubMed] [Google Scholar]
- Kumagai H, Sejima S, Choi Y, Tanaka H, Yamada H. (1975) Crystallization and properties of cysteine desulfhydrase from Aerobacter aerogenes. FEBS Lett 52:304–307. [PubMed] [Google Scholar]
- Lane P, Hao G, Gross SS. (2001) S-nitrosylation is emerging as a specific and fundamental posttranslational protein modification: head-to-head comparison with O-phosphorylation. Sci STKE 2001:re1. [PubMed] [Google Scholar]
- Lee E. (2016) Effects of nitric oxide on carotid intima media thickness: a pilot study. Altern Ther Health Med 22(Suppl 2):32–34. [PubMed] [Google Scholar]
- Lee J, Kim HT, Solares GJ, Kim K, Ding Z, Ivy JL. (2015) Caffeinated nitric oxide-releasing lozenge improves cycling time trial performance. Int J Sports Med 36:107–112. [PubMed] [Google Scholar]
- Levitt MD, Furne J, Springfield J, Suarez F, DeMaster E. (1999) Detoxification of hydrogen sulfide and methanethiol in the cecal mucosa. J Clin Invest 104:1107–1114. [PMC free article] [PubMed] [Google Scholar]
- Levitt MD, Springfield J, Furne J, Koenig T, Suarez FL. (2002) Physiology of sulfide in the rat colon: use of bismuth to assess colonic sulfide production. J Appl Physiol (1985) 92:1655–1660. [PubMed] [Google Scholar]
- Lundberg JO, Weitzberg E, Cole JA, Benjamin N. (2004) Nitrate, bacteria and human health. Nat Rev Microbiol 2:593–602. [PubMed] [Google Scholar]
- Morris MS, Picciano MF, Jacques PF, Selhub J. (2008) Plasma pyridoxal 5′-phosphate in the US population: the National Health and Nutrition Examination Survey, 2003-2004. Am J Clin Nutr 87:1446–1454. [PubMed] [Google Scholar]
- Motta JP, Flannigan KL, Agbor TA, Beatty JK, Blackler RW, Workentine ML, Da Silva GJ, Wang R, Buret AG, Wallace JL. (2015) Hydrogen sulfide protects from colitis and restores intestinal microbiota biofilm and mucus production. Inflamm Bowel Dis 21:1006–1017. [PubMed] [Google Scholar]
- Nagamani SC, Campeau PM, Shchelochkov OA, Premkumar MH, Guse K, Brunetti-Pierri N, Chen Y, Sun Q, Tang Y, Palmer D, et al. (2012) Nitric-oxide supplementation for treatment of long-term complications in argininosuccinic aciduria. Am J Hum Genet 90:836–846. [PMC free article] [PubMed] [Google Scholar]
- Paul BD, Snyder SH. (2015) H2S: a novel gasotransmitter that signals by sulfhydration. Trends Biochem Sci 40:687–700. [PMC free article] [PubMed] [Google Scholar]
- Pietri R, Román-Morales E, López-Garriga J. (2011) Hydrogen sulfide and hemeproteins: knowledge and mysteries. Antioxid Redox Signal 15:393–404. [PMC free article] [PubMed] [Google Scholar]
- Polhemus DJ, Lefer DJ. (2014) Emergence of hydrogen sulfide as an endogenous gaseous signaling molecule in cardiovascular disease. Circ Res 114:730–737. [PMC free article] [PubMed] [Google Scholar]
- Predmore BL, Kondo K, Bhushan S, Zlatopolsky MA, King AL, Aragon JP, Grinsfelder DB, Condit ME, Lefer DJ. (2012) The polysulfide diallyl trisulfide protects the ischemic myocardium by preservation of endogenous hydrogen sulfide and increasing nitric oxide bioavailability. Am J Physiol Heart Circ Physiol 302:H2410–H2418. [PMC free article] [PubMed] [Google Scholar]
- Rabus R, Venceslau SS, Wöhlbrand L, Voordouw G, Wall JD, Pereira IA. (2015) A post-genomic view of the ecophysiology, catabolism and biotechnological relevance of sulphate-reducing prokaryotes. Adv Microb Physiol 66:55–321. [PubMed] [Google Scholar]
- Rey FE, Gonzalez MD, Cheng J, Wu M, Ahern PP, Gordon JI. (2013) Metabolic niche of a prominent sulfate-reducing human gut bacterium. Proc Natl Acad Sci USA 110:13582–13587. [PMC free article] [PubMed] [Google Scholar]
- Roediger WEW, Duncan A, Kapaniris O, Millard S. (1993) Sulphide impairment of substrate oxidation in rat colonocytes: a biochemical basis for ulcerative colitis? Clin Sci (Lond) 85:623–627. [PubMed] [Google Scholar]
- Shen X, Carlström M, Borniquel S, Jädert C, Kevil CG, Lundberg JO. (2013) Microbial regulation of host hydrogen sulfide bioavailability and metabolism. Free Radic Biol Med 60:195–200. [PMC free article] [PubMed] [Google Scholar]
- Stokes KY, Dugas TR, Tang Y, Garg H, Guidry E, Bryan NS. (2009) Dietary nitrite prevents hypercholesterolemic microvascular inflammation and reverses endothelial dysfunction. Am J Physiol Heart Circ Physiol 296:H1281–H1288. [PubMed] [Google Scholar]
- Tang Y, Garg H, Geng YJ, Bryan NS. (2009) Nitric oxide bioactivity of traditional Chinese medicines used for cardiovascular indications. Free Radic Biol Med 47:835–840. [PubMed] [Google Scholar]
- Tayeh MA, Marletta MA. (1989) Macrophage oxidation of L-arginine to nitric oxide, nitrite, and nitrate: tetrahydrobiopterin is required as a cofactor. J Biol Chem 264:19654–19658. [PubMed] [Google Scholar]
- Tomasova L, Dobrowolski L, Jurkowska H, Wróbel M, Huc T, Ondrias K, Ostaszewski R, Ufnal M. (2016a) Intracolonic hydrogen sulfide lowers blood pressure in rats. Nitric Oxide 60:50–58. [PubMed] [Google Scholar]
- Tomasova L, Konopelski P, Ufnal M. (2016b) Gut bacteria and hydrogen sulfide: the new old players in circulatory system homeostasis. Molecules 21:1–18. [PMC free article] [PubMed] [Google Scholar]
- Wallace JL. (2010) Physiological and pathophysiological roles of hydrogen sulfide in the gastrointestinal tract. Antioxid Redox Signal 12:1125–1133. [PubMed] [Google Scholar]
- Woessner M, Smoliga JM, Tarzia B, Stabler T, Van Bruggen M, Allen JD. (2016) A stepwise reduction in plasma and salivary nitrite with increasing strengths of mouthwash following a dietary nitrate load. Nitric Oxide 54:1–7. [PubMed] [Google Scholar]
- Xia M, Chen L, Muh RW, Li PL, Li N. (2009) Production and actions of hydrogen sulfide, a novel gaseous bioactive substance, in the kidneys. J Pharmacol Exp Ther 329:1056–1062. [PMC free article] [PubMed] [Google Scholar]
- Zand J, Lanza F, Garg HK, Bryan NS. (2011) All-natural nitrite and nitrate containing dietary supplement promotes nitric oxide production and reduces triglycerides in humans. Nutr Res 31:262–269. [PubMed] [Google Scholar]